
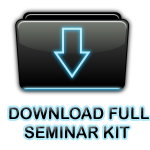
Video is now making the same conversion to digital technology for acquisition, storage, and communication. Witness the development of digital CCD cameras for image acquisition, digital transmission of TV signals (DBS), and video compression techniques for more efficient transmission, higher density storage on a video CD, or for video conference calls. The natural interface to digital video would be a digital display. But until recently, this possibility seemed as remote as developing a digital loudspeaker to interface with digital audio. Now there is a new MEMS-based projection display technology called Digital Light Processing (DLP) that accepts digital video and transmits to the eye a burst of digital light pulses that the eye interprets as a color analog image.
Digital Light Processing technology provides all digital projection displays that offer superior picture quality in terms of resolution, brightness, contrast, and color fidelity. This paper provides an overview of the digital light processing that have been developed by Texas Instruments for the all-digital display.
1.1 INTRODUCTION TO DLP:
Digital Light Processing is a revolutionary new way to project and display information. Based on the Digital Micro mirror Device developed by Texas Instruments, DLP creates the final link to display digital visual information. DLP technology is being provided as subsystems or "engines" to market leaders in the consumer, business, and professional segments of the projection display industry. In the same way the compact disc revolutionized the audio industry, DLP will revolutionize video projection.
DLP has three key advantages over existing projection technologies. The inherent digital nature of DLP enables noise-free, precise image quality with digital gray scale and color reproduction. Its digital nature also positions DLP to be the final link in the digital video infrastructure. DLP is more efficient than competing transmissive liquid crystal display (LCD) technology because it is based on the reflective DMD and does not require polarized light. Finally, close spacing of the micro mirrors causes video images to be projected as seamless pictures with higher perceived resolution. For movie projection, a computer slide presentation, or an interactive, multi-person, worldwide collaboration—DLP is the only choice for digital visual communications, today and in the future.
The world is rapidly moving to an all-digital communications and entertainment infrastructure. DMD and DLP technologies are introduced in the context of that infrastructure.
1.2 INTRODUCTION TO DMD:
Figure 1 The DMD microchip lies at the heart of the DLP system. It consists of an array of digital light Switches that accept electrical words as their inputs and output optical words. Surrounding the DMD are the necessary functionalities to take a digital source and project its undegraded image to a projection screen or hardcopy surface. These functionalities include image processing, memory, Reformatting, timing control, a light source, and projection optics. The input to the DLP system is a digital source (e.g., from a computer or DBS satellite receiver) or it may be NTSC video converted to digital.
Figure2: DLP PROCESSING SYSTEM
The basic building block of DLP technology is the DMD pixel, a reflective digital light switch. It is the equivalent of the electrical switch or gate in memory or microprocessor technologies. Unlike its electrical counterpart, however, the DMD light switch involves not only the electrical domain but also the mechanical and optical domains. Responding to an electrical input signal, the DMD light switch uses electromechanical action to interact with incident light and to switch that light into time-modulated light bundles at its output. This switching scheme is called binary pulse width modulation and is used to produce the sensation of gray scale to the observer's eye.
In the near future, most of the technologies necessary to achieve an all-digital communications and entertainment infrastructure will be available at the right performance and price levels. This will make an all-digital infrastructure chain such as the one shown in Figure 3commercially viable. The All-Digital Infrastructure
The links in this chain include capture, compression, transmission, reception, decompression, hearing, and viewing. But the final link is missing-an all-digital display. Digital images received today must be translated into analog signals for viewing on today's analog televisions. The digital display block shown in Figure 1 accepts a digital signal, but unlike analog displays of today, it outputs to the eye of the viewer an optical signal that is also digital. The viewer Perceives the digital signal as an analog signal, in essence performing the digital-to-analog (D/A) Conversion physiologically. An all-digital display possesses a degree of image stability and noise immunity that is inherently attributable to its digital nature.[1] Consider a digital word that is input electronically to the display. That word is converted into an optical word that is nearly immune to environmental, aging and manufacturing influences. DLP provides the all-digital projection display solution, accepting a digital electrical input and outputting a digital optical image. Figure 2 shows the functional elements of such a system. The Missing Link in the All-Digital Infrastructure
DMD Architecture
The world is rapidly moving to an all-digital communications and entertainment infrastructure.
DMD and DLP technologies are introduced in the context of that infrastructure.
2.1 The Mirror as a Switch
The address circuit and electromechanical superstructure of each pixel support one simple function, the fast and precise rotation of an aluminum micro mirror, 16 μm square, through angles of +10 and p;10 degrees. Figure 4 illustrates the architecture of one pixel, showing the mirror as semitransparent so that the structure underneath can be observed.
The schematic diagram of Figure 5 illustrates the optical switching action of the mirror. When the mirror rotates to its on state (+10 degrees), light from a projection source is directed into the pupil of a projection lens and the pixel appears bright on a projection screen. When the mirror rotates to its off state (-10 degrees), light is directed out of the pupil of the projection lens and the pixel appears dark. Thus, the optical switching function is simply the rapid directing of light into or out of the pupil of the projection lens.
2.2 DMD Cell Structure
To provide a thorough understanding of the DMD pixel structure and how it is addressed, we employ several figures involving exploded views, cutaway views, and an electrical schematic diagram. Figure 6shows a progressive cutaway view of a 3 x 3 array of pixels. Figure 7depicts how each layer is electrically interconnected and defines the bias and address voltages that must be applied to the pixel for proper switching action. The DMD pixel is a monolithically integrated MEMS superstructure cell fabricated over a CMOS SRAM cell. Plasma etching a sacrificial layer develops air gaps between the metal layers of the superstructure. The air gaps free the structure to rotate about two compliant torsion hinges. The mirror is connected to an underlying yoke which in turn is suspended by two thin torsion hinges to support posts. The yoke is electrostatically attracted to the underlying yoke address electrodes. The mirror is electrostatically attracted to mirror address electrodes. The mirror and yoke rotate until the yoke comes to rest against mechanical stops that are at the same potential as the yoke. The position of the mechanical stops limits the mirror rotation angle to +10 or -10 degrees.
The state of the SRAM cell (1,0) determines which mirror rotation angle is selected. Because geometry determines the rotation angle, as opposed to a balance of electrostatic torques as in earlier TI devices, the rotation angle of +10 or -10 degrees is precisely determined. The digital nature of the rotation angle guarantees high degree of brightness uniformity.
The address electrodes for the mirror and yoke are connected to the complementary sides of the underlying SRAM cell. The yoke and mirror are connected to a bias bus fabricated at the Metal- 3 layer. The bias bus interconnects the yoke and mirrors of each pixel to a bond pad at the chip perimeter. An off-chip driver supplies the bias waveform necessary for proper digital operation. The DMD mirrors are 16 μm square. They are arrayed to form a matrix having a high fill factor (approximately 90%) for maximum use of light.
2.3 Digital Nature of the DMD
The DMD pixel is operated in an electrostatically bistable mode to minimize the address voltage requirements. In this manner, we can achieve large rotation angles with conventional 5 volt CMOS devices. To this end, a bias voltage is applied to the yoke and mirror,for a mirror that is in its flat or quiescent state, the bias produces no net torque.
2.4 The Address Sequence
When the yoke and mirror flex, energy is stored as potential energy. When the reset pulse is turned off, the potential energy is converted into kinetic energy as the yoke and mirror unflex. Thus, the yoke landing tip is accelerated away from the landing pad to a distance sufficient for the hinge to take over and reliably return the mirror to its flat state.
The address sequence to be performed once each bit time can be summarized as follows:
1. Reset all mirrors in the array.
2. Turn off bias to allow mirrors to begin to rotate to flat state.
3. Turn bias on to enable mirrors to rotate to addressed states (+10/-10 degrees).
4. Keep bias on to latch mirrors (they will not respond to new address states).
5. Address SRAM array under the mirrors, one line at a time.
6. Repeat sequence beginning at step 1.
2.5 Evolution of the DMD Architecture
The cell structure shown in Figures 6 and 7 is the latest in a series of architectural changes that have improved the optical performance and reliability of the DMD digital light switch The basic bistable concept was developed in the Central Research Laboratories of Texas Instruments (now Corporate Research & Development). The first structure, known as the conventional pixel, did not hide the mechanical structures of the hinges or the support posts. This resulted in less area available for the mirror and greater light diffraction from the exposed mechanical structures. The result was a contrast ratio and optical efficiency that could not support a commercial business.
The first improvement made by the newly formed Digital Imaging Venture Project of Texas Instruments was to hide the hinges and support posts under the mirror (Hidden Hinge 1). This modification resulted in a greater mirror area and less light diffraction with an attendant improvement in contrast ratio (>100:1) and greater optical efficiency. But this structure could not work reliably with 5 volt CMOS levels. Two more superstructure designs were required before reliable operation was achieved. The current structure (Hidden Hinge 3) maximizes the available area for electrostatic attraction, using both the yoke and mirror as active elements. Thus, almost every bit of area is used to develop electrostatic torque, resulting in greater electrical efficiency
and reliability.
3. PROJECTION OPERATION
3.1 DMD Optical Switching Principle
The optical switching principle is briefly discussed in Section 2.1 and illustrated in Figure 5. Light from a projection source illuminates the DMD array at an angle of +2qL from the normal to the plane of mirrors in their flat state. The angle qL is the rotation angle of the mirror when the yoke is touching its mechanical stops, or landed. The mirror in its flat state reflects the incident light to an angle of -2qL. Because of the large rotation angles of the mirror, the off-state light and on-state light are widely separated, allowing fast projection optics to be used. The result is efficient light collection while maintaining a high contrast ratio.
3.2 Gray Scale
As previously mentioned, the DMD accepts electrical words representing gray levels of brightness at its input and outputs optical words. Suppose, for the sake of simplicity, that the input words have 4 bits, as shown in Figure 9
The possible gray levels produced by all combinations of bits in the 4-bit word are (2)4 or 16 equally spaced gray levels (0, 1/15, 2/15, , 15/15). For example, (0000) = 0, (1000) = 8/15, and (1111) = 15/15. The DMD commonly uses 8-bit words, representing (2)8 or 256 possible gray levels. Figure 9 is an example of binary pulse width modulation. In this simple example, the DMD array is illuminated with constant intensity light (not shown) and only 4-bit words are input to the array, representing 16 possible gray levels. A projection lens focuses and magnifies the light reflected from each pixel onto a distant projection screen. For clarity, only the central column is addressed. It is assumed that the others are addressed to the dark state (0000). An electrical word is input into the memory element of each light switch one bit at a time, beginning with the MSB for each word.
When the entire array of light switches has been addressed with the MSB, the individual pixels are enabled (reset) so that they can respond in parallel to their MSB state (1 or 0). During each bit time, the next bit is loaded into the memory array. At the end of each bit time, the pixels are reset and they respond in parallel to the next address bit. The process is repeated until all address bits are loaded into memory. Incident light is reflected from the light switches and is switched or modulated into light bundles having durations represented by each bit in the electrical word. To an observer, the light bundles occur over such a small time compared to the integration time of the eye that they give the physical sensation of light having a constant intensity represented by the value of the 4-bit input word.
Table 1. Distinguishing features of DMD MEMS technology
Number of moving parts | 0.5 to 1.2 million |
Mechanical motion | Makes discrete contacts or Landings |
Lifetime requirement | 450 billion contacts per moving part |
Address voltage | Limited by 5-volt CMOS Technology |
Mechanical elements | Aluminum Low temperature, sputter deposition, plasma etch |
Die separation | After removal of sacrificial Spacer |
Testing | High-speed electro-optical before die separation |
Sacrificial layer | Organic, dry-etched, wafer level removal |
DLP is accurate reproduction several combinations of different gray scale levels for each of the primary colors and the resultant digitally created of gray scale and color levels. The video infrastructure. DLP offers the final link to a complete digital video infrastructure. Another digital advantage is DLP as accurate reproduction of gray scale and color levels. And because each video or graphics frame is generated by a digital, 8- to 10-bits-per-color gray scale, the exact digital picture can be recreated time and time again
3.3 Projection Systems
The choice of how many DMD chips (one, two, or three) to employ in a DLP projection system is determined by the tradeoff between cost, light efficiency, power dissipation, weight, and volume. Figure 15 is an example of a single -chip DLP projection system. The light source is usually Metal halide because of its greater luminous efficiency (lumens delivered per electrical watt dissipated). A condenser lens collects the light, which is imaged onto the surface of a transmissive color wheel. A second lens collects the light that passes through the color wheel and evenly illuminates the surface of the DMD. Depending on the rotational state of the mirror (+10 or -10 degrees), the light is directed either into the pupil of the projection lens (on) or away from the pupil of the projection lens (off). The projection lens has two functions: (1) to collect the light from each on-state mirror, and (2) to project an enlarged image of the mirror surface to a Projection screen.
Figure 12 shows a front-projection display image. The field of view of the projection lens has been increased to show the chip perimeter, including the bond pads and wires. Normally, the chip perimeter has a light shield over it so that the display area is surrounded by a black background.
4. FABRICATION
The DMD superstructure is monolithically fabricated over an SRAM address circuit by conventional semiconductor processing techniques, including the steps of sputter metal deposit ion, lithography, and plasma etching. Plasma etching of an organic sacrificial layer forms the air gap. This sacrificial layer is simply a conventional, hardened positive photo resist layer. However, several of the packaging and testing steps in the fabrication flow differ significantly from those in a conventional CMOS wafer flow. These differences arise because of the mechanical and optical nature of the product.
We stated earlier that one of the remarkable aspects of DLP technology is the timely convergence of market needs and technology advances resulting in significant business opportunities for this all-digital projection display technology. The market needs for DLP technology fall into two general categories: displays and hard copy.
6. HARD COPY IN EVALUATION
Texas Instruments interest in the hardcopy application for DMD was the early driver for this unique technology. However, the brightness uniformity of the analog DMD was not sufficient to warrant its commercial use in a hardcopy application. Not until the invention of the digital DMD in 1987 could the companies seriously consider the DMD for hardcopy applications. Currently, TI has developed a 7056 x 64 hardcopy chip that is capable of projecting a 600 dpi resolution image over a print width of 11.7 inches (297 mm). Figure 13 the optical subsystem. The optical switching principle presented in Section 2.1 is common to both hardcopy and display applications
DLP, by its digital nature, requires the viewer's eye to perceive the digital output as an analog image. In a display application, optical words are imaged directly onto the retina of the eye where the viewer perceives these optical words as gray scale. For hardcopy applications, however, optical words are strobed as images onto an OPC drum, where they are integrated to produce a latent gray scale electrostatic image. The technique is called time integrate gray scale or TIG. The printer shown in Figure 13 has demonstrated near photographic quality images. Evaluations of DLP-based printing are continuing, with an eye toward product introductions in early 1997. .
7. The Digital Advantages
The audio world started the trend toward digital technology well over a decade ago. Recently, an abundance of new digital video technology has been introduced to the entertainment and communications markets.. The digital versatile disc (DVD), a widely anticipated new storage medium, will feature full-length films with better than laser disc video quality by placing up to 17 gigabytes of information on a single disc.
The Reflective Advantage
Because the DMD is a reflective device, it has a light efficiency of greater than 60%, making DLP systems more efficient than LCD projection displays. This efficiency is the product of reflectivity; fill factor, diffraction efficiency, and actual mirror "on" time.LCDs are polarization-dependent, so one of the polarized light components is not used. This means that 50% of the lamp light never even gets to the LCD because it is filtered out by a polarizer. Other light is blocked by the transistors, gate, and source lines in the LCD cell. In addition to these light losses, the liquid crystal material itself absorbs a portion of the light. The result is that only a small amount of the incident light is transmitted through the LCD panel and onto the screen. Recently,
Picture Advantage
The square mirrors on DMDs are 16 µm², separated by 1 µm gaps, giving a fill factor of up to 90%. In other words, 90% of the pixel/mirror area can actively reflect light to create a projected image. Pixel size and gap uniformity are maintained over the entire array and are independent of resolution. LCDs have, at best, a 70% fill factor.
8.0 Summary
The convergence of market needs and technology advances has created a unique business opportunity for an all-digital display technology based on the Digital Micro mirror Device (DMD). This paper presents an overview of this important new technology in terms of its architecture, projection operation, fabrication, and reliability. Digital Light Processing (DLP) systems incorporating the DMD are being developed for projection displays and hardcopy applications. Hardcopy systems using DLP are in an evaluation phase, with promising, near photographic quality printing having already been demonstrated. DLP-based projection display systems have been demonstrated in a variety of sizes and form factors. By the end of 1995, the first projection displays based on DLP will be available on the market.
Conclusion
Simply put, DLP is an optical system driven by digital electronics. The digital electronics and optics converge at the DMD. Using a video or graphics input signal, DLP creates a digitally projected image with unprecedented picture quality.
DLP has three key advantages over existing projection technologies. The digital nature of DLP enables digital gray scale and color reproduction and also positions DLP to be the final link in the digital video infrastructure. Because it is based on the reflective DMD, DLP is more efficient than competing transmissive LCD technologies. Finally, DLP has the ability to create seamless, film like images. DLP makes images look better. You've heard the digital revolution, now see it with Digital Light Processing.
REFERENCES
1. R.J. Gove, "DMD Display Systems: The Impact of an All-Digital Display," Society for Information Display International Symposium (June 1994).
2.L.J. Hornbeck and W.E. Nelson, "Bistable Deformable Mirror Device," OSA Technical Digest Series Vol. 8, Spatial Light Modulators and Applications, p. 107 (1988).
3.L.J. Hornbeck, "Deformable-Mirror Spatial Light Modulators," Spatial Light Modulators and Applications III, SPIE Critical Reviews, Vol. 1150, pp. 86-102 (August 1989).
4.W.E. Nelson, L.J. Hornbeck, Micromechanical Spatial Light Modulator for Electro photographic Printers, SPSE Fourth International Congress on Advances in Non-Impact Printing Technologies, p. 427, March 20, 1988.
5. J.B. Sampsell, "An Overview of Texas Instruments Digital Micro mirror Device (DMD) and Its Application to Projection Displays," Society for Information Display Internatl. Symposium Digest of Tech. Papers, Vol. 24, pp. 1012-1015 (May 1993).
6.L.J. Hornbeck, "Current Status of the Digital Micro mirror Device (DMD) for Projection Television Applications (Invited Paper)," International Electron Devices Technical Digest, pp. 381-384 (1993).
7. J.M Younse and D.W. Monk, "The Digital Micro mirror Device (DMD) and Its Transition
to HDTV," Proc. of 13th International Display Research Conf. (Late News Papers), pp.
613-616 (August 31-September 3, 1993).
8. J.B. Sampsell, "The Digital Micro mirror Device," 7th ICSS&A, Yokohama, Japan (1993).
9. J.M. Younse, "Mirrors on a Chip," IEEE Spectrum, pp. 27-31 (November 1993).
10. M.A. Mignardi, "Digital Micro mirror Array for Projection TV," Solid State Technology,
Vol. 37, pp. 63-66 (July 1994).
No comments:
Post a Comment
leave your opinion