
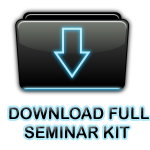
This report presents an overview of techniques for visualization of fluid flow data.
The popular techniques Temperature Sensitive Paint, Pressure Sensitive Paint, Tuft Method, Hydrogen Bubble, Optical Methods, and Particle Imaging Velocimetry are explained in details. The figures for various techniques have been presented at the end of this report.
This report mainly concentrated on the experimental fluid flow visualization. Still important computer aided visualization methods have summarized, like the particle imaging Velocimetry. A summary of all the important techniques have been presented at the end of the report. Introduction to the computer graphics flow visualization have been made so that the reader can have a basic idea of the techniques of graphics visualization.
Purposes and Problems of Flow Visualization
Flow visualization probably exists as long as fluid flow researches itself. Until recently,
Experimental flow visualization has been the main visualization aid in fluid flow research. Experimental flow visualization techniques are applied for several reasons:
• To get an impression of fluid flow around a scale model of a real object, without any
Calculations;
• As a source of inspiration for the development of new and better theories of fluid flow;
• To verify a new theory or model.
Though used extensively, these methods suffer from some problems. A fluid flow is often affected by the experimental technique, and not all fluid flow phenomena or relevant parameters can be visualized with experimental techniques. Also, the construction of small scale physical models and experimental equipment such as wind tunnels are expensive, and experiments are time consuming.
Recently a new type of visualization has emerged: computer-aided visualization. The increase of computational power has led to an increasing use of computers for numerical simulations. In the area of fluid dynamics, computers are extensively used to calculate velocity fields and other flow quantities, using numerical techniques to solve the governing Navier-Stokes equations. This has led to the emergence of Computational Fluid Dynamics (CFD) as a new field of research and practice.
To analyze the results of the complex calculations, computer visualization techniques are necessary. Humans are capable of understanding much more information when it is shown visually, rather than numerically. By using the computer not only for calculating the numerical data, but also for visualizing these data in an understandable way, the benefits of the increasing computational power are much greater.
The visualization of fluid flow simulation data may have several different purposes. One purpose is the verification of theoretical models in fundamental research. When a flow phenomenon is described by a model, this flow model should be compared with the ‘real’ fluid flow. The accuracy of the model can be verified by calculation and visualization of a flow with the model, and comparison of the results with experimental results. If the numerical results and the experimental flow are visualized in the same way, a qualitative verification by visual inspection can be very effective. Research in numerical methods for solving the flow equations can be supported. By visualizing the solutions found, but also by visualization of intermediate results during the iterative solution process.
Another purpose of fluid flow visualization is the analysis and evaluation of a design. For the design of a car, an aircraft, a harbor, or any other object that is functionally related with fluid flow, calculation and visualization of the fluid flow phenomena can be a powerful tool in design optimization and evaluation. In this type of applied research, communication of flow analysis results to others, including non-specialists, is important in the decision making process.
In practice, often both experimental and computer-aided visualization will be applied. Fluid flow visualization using computer graphics will be inspired by experimental visualization. Following the development of 3D flow solution techniques, there is especially an urgent need for visualization of 3D flow patterns. This presents many interesting but still unsolved problems to computer graphics research. Flow data are different in many respects from the objects and surfaces traditionally displayed by 3D computer graphics. New techniques are emerging for generating informative images of flow patterns; also, techniques are being developed to transform the flow visualization problem to display of traditional graphics primitives.
Experimental Flow Visualization
1. Pressure Sensitive Paint (psp) And Temperature Sensitive Paint (tsp)
The use of luminescent molecular probes for measuring surface temperature and pressure on wind tunnel models and flight vehicles offers the promise of enhanced spatial resolution and lower costs compared to traditional techniques. These new sensors are called temperature-sensitive paint (TSP) and pressure-sensitive paint (PSP).
Traditionally, arrays of thermocouples and pressure taps have been used to obtain surface temperature and pressure distributions. These techniques can be very labor-intensive and model/flight vehicle preparation costs are high when detailed maps of temperature and pressure are desired. Further, the spatial resolution is limited by the number of instrumentation locations chosen. By comparison, the TSP and PSP techniques provide a way to obtain simple, inexpensive, full-field measurements of temperature and pressure with much higher spatial resolution. Both TSP and PSP incorporate luminescent molecules in a paint which can be applied to any aerodynamic model surface. Figure 1 shows a schematic of a paint layer incorporating a luminescent molecule.
The paint layer is composed of luminescent molecules and a polymer binder material. The resulting ‘paint’ can be applied to a surface using a brush or sprayer. As the paint dries, the solvent evaporates and leaves behind a polymer matrix with luminescent molecules embedded in it. Light of the proper wavelength to excite the luminescent molecules in the paint is directed at the model and luminescent light of a longer wavelength is emitted by the molecules Using the proper filters, the excitation light and luminescent emission light can be separated and the intensity of the luminescent light can be determined using a photo detector. Through the photo-physical processes known as thermal- and oxygen quenching, the luminescent intensity of the paint emission is related to temperature or pressure. Hence, from the detected luminescent intensity, temperature and pressure can be determined. The polymer binder is an important ingredient of a luminescent paint used to adhere the paint to the surface of interest. In some cases, the polymer matrix is a passive anchor. In other cases, however, the polymer may affect significantly the photo physical behavior of the paint through a complicated interaction between the luminescent molecules and the macro-molecules of the polymer. A good polymer binder should be robust enough to sustain skin friction and other forces on the surface of an aerodynamic model. Also, it must be easy to apply and repair to the surface in a smooth, thin film.
For TSP, many commercially available resins and epoxies can be chosen serve as polymer binders if they are not oxygen permeable and do not degrade the activity of the luminophore molecules. In contrast, a good polymer binder for a PSP must have high oxygen permeability besides being robust and easy to apply. The CCD camera system for luminescent paints is the most commonly used in aerodynamic testing. A schematic of this system is shown in Figure 2. The luminescent
Paint (TSP or PSP) is coated on the surface of the model. The paint is excited to
Luminance by the illumination source, such as a lamp or a laser. The luminescent intensity Image is filtered optically to eliminate the illuminating light and then captured by a CCD
Camera and transferred to a computer with a frame grabber board for image processing.
Both wind-on image (at temperature and pressure to be determined) and wind-off image
(At a known constant temperature and pressure) are obtained. The ratio between the wind on and wind-off images is taken after the dark current level image is subtracted from both
Images, yielding a relative luminescent intensity image. Using the calibration relations, the surface temperature and pressure distributions can be computed from the relative
Luminescent intensity image.
TSP has also been utilized as an approach to flow transition detection since convective heat transfer is much higher in turbulent flow than in laminar flow, TSP can visualize the surface temperature difference between turbulent and laminar regions. In low speed wind tunnel tests, the model is typically heated or cooled to enhance temperature variation across the transition line.
The PSP/TSP technique provides a promising tool for measuring surface pressure
distributions on a high-speed rotating blade at a high spatial resolution. Instrumentation is
Particularly difficult in the rotating environment and the pressure taps weaken the
Structure of the rotating blade. Recently, a test was performed to measure the chord wise pressure distributions on the rotor blades of a high speed axial flow compressor/ TSP (Ru(bpy)-Shellac) and PSP (Ru(ph2-phen) in GE RTV 118) were applied to alternating blades. The TSP provided the temperature distributions on the blades for
temperature correction of the PSP results. A scanning laser system was used for
excitation and detection of luminescence. Both the TSP and PSP were excited with an
Argon laser and luminescence was detected with a Hamamatsu PMT. The same system
was used on an Allied Signal F109 gas turbine engine giving the suction surface
pressure map at 14000 rpm shown in Figure 3
As previously mentioned, pressure sensitive paints are used to measure surface pressures. The conventional methods of measuring these pressures are to apply pressure taps or transducers to a model, but these approaches have some significant disadvantages.
First of all, taps and transducers only allow measurements at discrete points on the model surface. The surface pressures at other locations on the model can only be interpolated from the known points. Another disadvantage is that taps and transducers are intrusive to the flow. Measurements cannot be taken downstream of other taps or transducers, since the flow is altered once it passes over the upstream disturbances. Finally, taps and transducers are time-consuming and expensive to use. Typical models used for determining surface loads in aircraft design typically cost $500,000 to $1 million, with approximately 30% of that cost going towards the pressure taps and their installation.
A relatively new method to surface pressure measurement utilizes pressure sensitive paint, or PSP. Pressure sensitive paint has numerous advantages over the more conventional pressure taps and transducers. The most obvious is that PSP is a field measurement, allowing for a surface pressure determination over the entire model, not just at discrete points. Hence, PSP provides a much greater spatial resolution than pressure taps, and disturbances in the flow are immediately observable.
PSP also has the advantage of being a non-intrusive technique. Use of PSP, for the most part, does not affect the flow around the model, allowing its use over the entire model surface. The use of PSP eliminates the need for a large number of pressure taps, which leads to more than one benefit. Since pressure taps do not need to be installed, models can be constructed in less time, and with less money than before. Also, since holes do not need to be drilled in the model for the installation of taps, the model strength is increased, and higher Reynolds numbers can be obtained. Not only does the PSP method reduce the cost of the model construction, but it also reduces the cost of the instrumentation needed for data collection. In addition, the equipment needed for PSP costs less than pressure taps, but it can also be easily reused for numerous models.
In aircraft design, PSP has the potential to save both time and money. The continuous data distribution on the model provided by PSP can easily be integrated over specific components, which can provide detailed surface loads. Since a model for use with the PSP technique is faster to construct, this allows for load data to be known much earlier in the design process.
Disadvantages:
Unfortunately, PSP is not without its undesirable characteristics. One of these characteristics is that the response of the luminescent molecules in the PSP coating degrades with time of exposure to the excitation illumination. This degradation occurs because of a photochemical reaction that occurs when the molecules are excited. Eventually, this degradation of the molecules determines the useful life of the PSP coating. This characteristic becomes more important for larger models, as the cost and time of PSP reapplication becomes a significant factor.
A second undesirable characteristic of PSP is that the emission intensity is affected by the local temperature. This behavior is due to the effect temperature has on the energy state of the luminescent molecules, and the oxygen permeability of the binder. This temperature dependence becomes even more significant in compressible flow tests, where the recovery temperature over the model surface is not uniform.
Experimental Setup
As seen below, the PSP experimental setup is composed of a number of separate elements. The specifications of each element are dependent upon the test conditions, objectives, and budget.
Advantages:
As previously mentioned, pressure sensitive paints are used to measure surface pressures. The conventional methods of measuring these pressures are to apply pressure taps or transducers to a model, but these approaches have some significant disadvantages.
First of all, taps and transducers only allow measurements at discrete points on the model surface. The surface pressures at other locations on the model can only be interpolated from the known points. Another disadvantage is that taps and transducers are intrusive to the flow. Measurements cannot be taken downstream of other taps or transducers, since the flow is altered once it passes over the upstream disturbances. Finally, taps and transducers are time-consuming and expensive to use. Typical models used for determining surface loads in aircraft design typically cost $500,000 to $1 million, with approximately 30% of that cost going towards the pressure taps and their installation.
A relatively new method to surface pressure measurement utilizes pressure sensitive paint, or PSP. Pressure sensitive paint has numerous advantages over the more conventional pressure taps and transducers. The most obvious is that PSP is a field measurement, allowing for a surface pressure determination over the entire model, not just at discrete points. Hence, PSP provides a much greater spatial resolution than pressure taps, and disturbances in the flow are immediately observable.
PSP also has the advantage of being a non-intrusive technique. Use of PSP, for the most part, does not affect the flow around the model, allowing its use over the entire model surface. The use of PSP eliminates the need for a large number of pressure taps, which leads to more than one benefit. Since pressure taps do not need to be installed, models can be constructed in less time, and with less money than before. Also, since holes do not need to be drilled in the model for the installation of taps, the model strength is increased, and higher Reynolds numbers can be obtained. Not only does the PSP method reduce the cost of the model construction, but it also reduces the cost of the instrumentation needed for data collection. In addition, the equipment needed for PSP costs less than pressure taps, but it can also be easily reused for numerous models.
In aircraft design, PSP has the potential to save both time and money. The continuous data distribution on the model provided by PSP can easily be integrated over specific components, which can provide detailed surface loads. Since a model for use with the PSP technique is faster to construct, this allows for load data to be known much earlier in the design process.
Disadvantages:
Unfortunately, PSP is not without its undesirable characteristics. One of these characteristics is that the response of the luminescent molecules in the PSP coating degrades with time of exposure to the excitation illumination. This degradation occurs because of a photochemical reaction that occurs when the molecules are excited. Eventually, this degradation of the molecules determines the useful life of the PSP coating. This characteristic becomes more important for larger models, as the cost and time of PSP reapplication becomes a significant factor.
A second undesirable characteristic of PSP is that the emission intensity is affected by the local temperature. This behavior is due to the effect temperature has on the energy state of the luminescent molecules, and the oxygen permeability of the binder. This temperature dependence becomes even more significant in compressible flow tests, where the recovery temperature over the model surface is not uniform.
Experimental Setup
As seen below, the PSP experimental setup is composed of a number of separate elements. The specifications of each element are dependent upon the test conditions, objectives, and budget.
Typical PSP experimental setup
Illumination:
The illumination element ("light source") of the setup is used to excite the luminescent molecules in the PSP coating. Since the intensity of the emitted illumination is proportional to the excitation illumination, the source of illumination must be of sufficient power in the absorption spectrum of the PSP coating, and also have a stable output over time. For complex models with numerous surfaces, multiple illumination elements are often needed to achieve an adequate coverage of the model surface. Some examples of illumination elements are lasers, continuous and flash arc lamps, and simple incandescent lamps.
Imaging:
The imaging element ("camera") used in the experimental setup is heavily dependent upon the required results. In most cases, a good spatial resolution of the pressure distribution is required. Imaging elements that can provide a good spatial resolution include conventional still photography, low-light video cameras, or scientific grade CCD cameras. In most PSP applications, the electronic CCD cameras are the preferred imaging element due to their good spatial resolution and capability to reduce the data they acquire in real time. CCD cameras can be divided into two groups, conventional black and white video cameras and scientific grade CCD digital cameras.
Conventional black and white video cameras are attractive mainly due to their low cost. Typical cameras deliver an 8-bit intensity resolution over a 640 X 480 pixel spatial resolution. Even though conventional black and white video cameras are not precision scientific instruments, when coupled with a PC image processor, the results obtained are more than acceptable for qualitative analysis, and are potentially acceptable for quantitative analysis in certain conditions.
Scientific grade cooled CCD digital cameras, on the other hand, are precision scientific instruments that provide high-precision measurements, at the price of an increased cost. Typical cameras of this type can exhibit 16-bit intensity resolution and spatial resolution up to 2048 X 2048 pixels. For many PSP applications, the high resolution provided by these cameras is mandatory. Optical Filters:
In order to avoid erroneous illumination readings, it is necessary that the illumination element only output in the absorption spectrum, while the imaging element only records the emission spectrum. When lasers are used for excitation purposes, this is not an issue, as a laser only produces light in one wavelength. Most excitation sources, however, produce light in a wide spectrum. In order to prevent the excitation source spectrum from overlapping the emission spectrum, optical filters are placed over both the illumination element and the imaging element. This constraint also makes it necessary to conduct all PSP testing in a darkened test section; otherwise ambient light may contaminate the readings.
Data Acquisition & Post Processing:
The data acquisition and post processing in most PSP applications is done in a modular fashion. Initially the camera and computer acquire images for wind-on and wind-off conditions. These images can then be corrected and processed as necessary, either on the same or different machine. This modular approach provides a benefit in that the processing for small-scale tests can easily be done with common software running on PCs. In larger-scale facilities, however, much more computing power is needed, as runs can easily produce large amounts of data that need to be processed. This leads to the requirement of high power graphics workstations and high capacity storage facilities. It is also important to note that in the false color is typically added to the images in the post-processing phase in order to facilitate flow visualization (PSP is monochromatic).
2.Liquid Films
This method makes use of the contrast obtained on account of the unequal rates of evaporation of a liquid film in the laminar and turbulent regions. A film of some volatile oil is applied on the surface of the model prior to starting of the flow. When the air flow takes place over this surface the evaporation of the oil film is faster in the turbulent than in the laminar region. A clearer contrast is obtained by using black paint on the surface. This method can be easily employed for Aeroflot blade surfaces in wind tunnels.
No comments:
Post a Comment
leave your opinion