
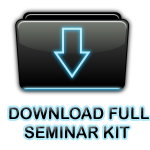
Skin cancers and tumors are an increasing problem around the world. Skin cancers account for about 40% of all the diagnosed cancers. Almost all skin cancers are curable, if detected early. Some cause morbidity if untreated. Currently, clinical dermatologists rely on visual inspection and experience to make an initial assessment of the skin lesion state. If further visual analysis does not provide a conclusive decision on the lesion state, such suspicious lesions are sent for biopsy analysis. Biopsy is unpleasant for the patient,slow in diagnostic results, and costly for the hospital on account of the wait time. Dermatologists would greatly benefit from a fast and noninvasive technique that could assist them in their clinical diagnostic decisions. Recent studies have suggested the close relationship between the various stages of skin diseases and the optical properties of the affected area. Finding the optical properties help a lot in the diagnosis. All the photo biological effects which are responsible for the variations in the optical properties of skin like the UV irradiation [which causes erythema (skin reddening), stimulates melanin synthesis, and even induce edema and tissue proliferation if the radiation dose is sufficiently large] need to be taken into consideration when prescribing phototherapy. So we need to find the optical properties of human skin.
OPTICAL PROPERTIES OF HUMAN SKIN
In terms of optical properties, biotissues (including blood, lymph, and other biological fluids) can be categorized into two large classes
v strongly scattering (opaque) tissues such
as skin, brain, vascular walls, blood, and
v weakly scattering (transparent) tissues such as the cornea and lens in the anterior eye chamber
Optical properties of human skin vary according to age and race. Biological tissues are optically inhomogeneous absorption media whose average refractive index is higher than that of air. This account for the partial reflection of radiation at the tissue/air interface (Fresnel reflection) while the remaining part penetrates the bio object. Multiple scattering and absorption are responsible for laser beam broadening and eventual decay as it travels through a biotissue whereas volume scattering is a major cause of the dispersion of a large fractionof radiation in the backward direction (backscattering).
Cellular organelles such as mitochondria are the main scatterers in various biotissues. Absorbed light is converted to heat and reradiated in the form of fluorescence; it is also consumed in photo-biochemical reactions. The absorption spectrum depends on the type of predominant absorption
centers and water content of biotissues.
DIFFERENT METHODS OF DETERMINING THE OPTICAL PROPERTIES
There are several methods to measure absorption and scattering properties by analyzing reflected or transmitted light.
v Steady-state spatial resolved
Steady-state spatially resolved measurements of a reflectance profile is taken from a point source or a narrow collimated light beam. This profile can be measured with a contact fiber optic probe. Measurements with fiber optic probes are simple and inexpensive, however, they require contact to the tissue, which can induce variations because the applied pressure affects local blood content in tissue.
v Time domain
Time domain is realized by means of the exposure of a scattering object to short laser pulses and the subsequent recording of scattered broadened pulses.This method is also called Time-Resolved method. The time-resolved regime is based on the excitation of the photon-density wave spectrum in a strongly scattering medium.
v Frequency domain
Frequency domain is realised by irradiation with modulated light in the frequency range 100 MHz to 10 GHz and recording the degree of modulation of scattered light intensity and the corresponding phase shift at modulation frequencies. This is also called phase method.
SOME TECHNIQUES FOR FINDING THE OPTICAL PROPERTIES
v Monte carlo method
The Monte Carlo method has recently been applied to biotissue optics.This method is based on the numerical simulation of photon transport in scattering media. Random migrations of photons inside a sample can be traced from their input till absorption or output. Known algorithms allow a few biotissue layers with different optical properties to be characterized along with the final incident beam size and the reflection of light at interfaces.
v Scanning fluorescence microscopy
Scanning fluorescence microscopy using two-photon excitation has become a powerful tool for performing high resolution imaging of optically dense material such as the human skin. For example two-photon microscopy (TPM) has been used for non-invasive skin cancer diagnostics and to study trans-dermal drug delivery .The human skin is, however, an optical challenge, due to its complex structure and varying optical properties. The cells of the different skin layers alter in size, shape and structure. The optical resolution is therefore not only dependent on the performance of the imaging system, e.g. the excitation wavelength, the numerical aperture of the objective, aberrations and misalign-ments, but also strongly on the properties of the skin.
v Integrating sphere method
In vitro evaluation is most often achieved by the double integrating sphere method combined with collimated transmittance measurements. This approach implies either sequential or simultaneous
determination of three parameters: collimated transmittance, diffuse transmittance Td, and diffuse reflectance Rd. The optical parameters of the tissue are deduced from these measurements using different theoretical expressions or numerical methods. The measurements were carried out using a commercially available spectrophotometer with an integrating sphere. The inverse adding–doubling method was used to determine the absorption and reduced scattering coefficients from the measurements.
v OIDR Spectrometry
Oblique Incidence Diffuse Reflectance Spectrometry is an optical method capable of quantifying both bulk absorption and scattering optical properties of heterogenous media by measuring the diffuse reflectance of the media.
DETERMINATION OF OPTICAL PROPERTIES USING STEADY STATE SPATIAL RESOLVED METHOD
In steady state spatial resolved method, we focus on the measurement of the escaping flux from a tissue at different distances from the source. The escaping flux is the diffuse reflecta-nce, which can be used to determine the optical properties. Oblique Incidence Diffuse Reflectance Spectrometry (OIDRS) can be used for measuring the diffuse reflectance of the media and is capable of quantifying both bulk absorption and scattering optical properties of heterogenous media. Diffuse reflectance is the probability of photon re-emission per unit surface area of a scattering medium.
Oblique-incidence DRS is used in a multifiber array mode such that spatial information is also gathered along tissue surface. We have combined the OIDRS technique with image processing and pattern recognition to provide the dermatologist with a computer-assisted tool to determine the state of unknown lesions in clinical settings.
PRINCIPLE OF OIDRS
When a light is incident on the surface of a heterogenous media (like skin), part of the incident light will be directly reflected, which is known as the specular reflectance and the remainder will transmit into and will interact with the media. After undergoing multiple times of scattering and absorption, part of the transmitted light will be turned back and will escape from the surface of the media, which forms the diffuse reflectance.
ADVANTAGES OF OIDRS
It is a fast and noninvasive technique, with oblique incidence, to identify benign and cancerous or cancer-prone skin lesions for the purpose of reducing unwanted benign biopsies. OIDRS has an advantage over normal incidence by gathering information from superficial layers without penetrat-ing deep tissue. This is of particular importance, since skin cancer information is usually present in the top layers of the skin tissue, and deeper layers only add to the background noise in the signal. In addition, oblique incidence DRS is sensitive to scattering and is able to separate absorption from scattering in homogeneous scattering media.
WORKING
The source light is delivered to the skin surface via a source fiber within a fiber-optic probe . The receiver fibers collect the diffusely reflected light from the skin surface and deliver the collected light to the spectrograph. The spectrograph disperses the one dimensional (1-D) light from each fiber then projected in the form of a two-dimensional (2-D) image onto the CCD matrix. The spectral images obtained from the CCD represents the steady state diffuse reflectance spectra from each receiver fiber.
The personal computer records the spectral images.We can obtain the spatially resolved steady state diffuse reflectance at a certain wavelength. From the diffuse reflectance, we can find the absorption coefficient and the reduced scattering coefficient.
EQUIPMENTS USED
The image acquisition for the OIDRS system is composed of a light source, transmission, source and receiver fibers, a spectrograph, a CCD camera, and a personal computer.
v Light source
The excitation or illumination light source is typically a laser or a filtered white light source, such as a xenon, halogen or mercury lamp for multiple wavelength measurement. Dielectric bandpass filters, monochromators, or double monochromators can be used as filters according to the requirements for spectral purity.
v Transmission, source & receiver fibers
Optical fibers have been the medium of choice in the field of optical communications for long distance propagation of light due to their excellent transmission characteristics and ability to be fabricated in lengths of many kilometers. Light propagates through the core region of optical fibers and these core regions can be as small as a few microns in diameter. Generally, an opto-mechanical switch is optically connected to the light conducting fibers and enables different communication paths to be interconnected.
Optical fibers, whether used in tele-communications or as environmental sensors, may include a cylindrical core, a concentric cylindrical cladding surrounding the core, and a concentric cylindrical protective jacket or buffer surrounding the cladding. The core is generally made of trans-parent glass or plastic having a certain index of refraction. The cladding is also made of transparent glass or plastic, but having a different, smaller, index of refraction. The ability of the optical fiber to act as a bendable waveguide is largely determined by the relative refractive indices of the core and the cladding.
v Fiber Optic Sensor Probe
The fiber-optic probe is made from brass tubing and 200m diameter, low-OH optical fibers. The optical fibers consist of 1source fiber and 13 receiver fibers. The source fiber is oriented at 45° with respect to the horizontal axis. The 13 vertical receiver fibers are arranged in a linear array spann-ing 3.5 mm. The receiver fibers collect the diffusely reflected light from the skin surface and deliver the collected light to the spectrograph. The probe is po-sitioned on the skin surface without pressure for image acquisition. The effective probe testing area is limited to 2*2 m2 to ensure that the measured area does not include the surrounding normal skin
even for the smallest skin lesions. Because the
OIDRS measurement is usually done in a dark environment to reduce the effect of the back ground light, some of the source fibers are used to illuminate the area of interest on the skin to ensure the accu-rate placement of the sensor probe. Many arrays of collection fibers are used for multiple data collect-ions from the same location on the skin to ensure a reliable and robust measurement.
To achieve accurate allignment of the collection fibers, two micro-machined fiber optic
devices were fabricated. A simple MEMS-compatible fabrication process to accomplish micromachining on the fiber end face has been developed.This sensor configuration significantly reduces the size of the sensor and makes the packaging simple and adhes-ive free. The micro-machining process of the present invention also provides for the fabrication of arrays of sensors that would provide two dimensional maps with high spatial resolution of at least one of acou-stical vibration, mechanical vibration, pressure, temperature, acceleration, electrostatic fields, magnetic fields or combinations thereof.
Each positioning device consists of a silicon substrate with linear array of v-grooves created with silicon bulk etching. When an optical fiber is placed in a v-groove, the center axis of the optical fiber can “automatically” align with the symmetric plane of the V-groove. Thus, the accurate positioning of each collection fiber in the array can be readily achieved to ensure reliable and uniform performance of the
sensor probe. To fabricate the positioning device, silicon nitride was deposited on a {100} silicon wafer. Photolithography and reactive ion etching was conducted to pattern the silicon nitride layer, which serves as a hard mask for silicon bulk etching. Silicon bulking etching was performed in potassium hydroxide solution to form the V-grooves.
For aligning the source fibers, two other micromachined positioning devices were fabricated. SU-8 resist was used for the fabrication of the guiding structures on a silicon substrate. SU-8 is
preferable for this process as it can be directly used to form structures over 1000µm in thickness, which results in a very simple and low-fabricationprocess. To fabricate the SU-8 guiding structure, a silicon substrate is first cleaned and baked at 200degree celsius for 5 min. SU-8 100 resist was spun on the cleaned silicon substrate at calibrated spinning rate
for 40s to reach a final thickness of about 100µm. A
soft bake was conducted at 65deg celsius for 10 min and then at 95 deg celsius for 30 min, which was followed by an ultraviolet(UV) exposure of a dosage of
about 520 mJ/cm. After the exposure the wafer was baked at 65 deg celsius for 1min and then at 95 deg celsius for 10min to selectively crosslink the exposed part of the SU-8 film. The development of exposed
SU-8 film was conducted for a few minutes until unexposed region was completely removed. During the SU-8 processing a slow temperature ramping was used to minimize the internal stress build up
and also the resulting crack formation within the
SU-8 film, which would significantly reduce the mechanical strength and stability of the guiding structures.
After the fabrication of the micropositioning device was complete, the entire OIDRS sensor probe was assembled. First, both the source and collection fibers were fixed into their own guiding structures. Because the thickness of the SU-8 guiding structure is 100µm, two positioning devices were placed face-to-face to accommodate the source fibers. For the collection fibers, one positioning devices were stacked and glued together with epoxy. To improve
the efficiency of incidence and collection of the fibers, the head of the assembled probe was polished with sand papers.During polishing care was taken to avoid any possible damage to the fibers. Finally, the assembled probe was placed in an aluminium probe holder to facilitate OIDRS testing.
v Spectrograph
The outputs from the receiver fibers are placed at the object plane of the imaging spectro-graph. The spectrograph disperses the one dimens-ional (1-D) light from each fiber into the spectral
components between 518 and 760 nm. The spectra from the 13 fibers are then projected in the form of a two-dimensional (2-D) image onto the CCD matrix.
v CCD camera
The CCD camera has a 512-512 pixel array of area 9.7mm-9.7mm. It collects spectral images
from the wavelength range of 455 to 765nm. The
spectral images represent the steady state diffuse reflectance spectra from each collection fiber.This system is capable of capturing one frame of spectral image in a fraction of second. The vertical dimension of the CCD array represents the spatial distribution of the diffuse reflectance in terms of the fiber locations. The horizontal dimension represents the
spectral distribution for the diffusely reflected light collected by each receiver fiber.
v Personal Computer
The personal computer controls the image acquisition parameters and records the spectral ima-ges automatically.
COLLECTED IMAGES OF in-vivo SKIN LESIONS
From the obtained spectral images, the absorption and scattering coefficients are calculated independently for each wavelength λ, using the corresponding diffuse reflectance along the x-axis.




The extinction coefficient which is the absorbance (of light) per unit path length and per unit of concentra-tion, can also be found out. The absorption coefficie-nt can be used to estimate important physiological parameters related to the diseased state such as the concentration of oxy-haemoglobin, deoxy-haemo-globin and its oxygen saturation. Extinction coefficients of melanin, oxy haemoglobin and deoxy haemoglobin are shown in fig.
Only the suspicious lesions, whose state the dermatologist could not determine by visual inspect-ion, are included in the sample pool. The images are subdivided into two types of cancer groups, namely, carcinomas- basal and squamous cell and melano-mas. The dermatologist is able to separate the two cancerous subgroups, or suspicious lesions belong-ing to these subgroups, by colour and other visual textural characteristics. The collected samples consist of benign keratoses, warts and cancerous squamous and basal cell carcinoma lesions and of benign common nevi and intermediate or potentially cancerous dysplastic nevi lesions.
For each image, the probe is removed and randomly re-positioned on the lesion or healthy skin surface to obtain multiple and minimally dependent images from different locations or orientations on the skin surface.
CONCLUSION
Optical properties of human skin vary according to age and race. Optical properties can be found out from the spectral images obtained fron the spectrophotometer . The dermatologist is able to identify the affected skin , by colour and other visual textural characteristics of the spectral images. From the spectral images, the absorption and scattering coefficients are calculated. The absorption coefficient can be used to estimate important physiological parameters related to the diseased state such as the concentration of oxy-haemoglobin, deoxy haemo-globin and its oxygen saturation. Thus the optical property of skin can be assessed.
REFERENCES
· “Determination of human skin optical properties in vivo from reflectance spectroscopic measurements”, Vol.5, No.3, Chinese Optics Letters.
· “Micromachined Fiber Optic sensor for in vivo measurement of optical properties of human skin”, Vol.8, No.10, IEEE Sensors Journal.
· “Determining the reduced scattering of skin
in-vivo using sized - fiber reflectometry”, paper
presented by T. P. Moffitt and S. A. Prahl,
ECE Dept., Oregon Health & Science University.
No comments:
Post a Comment
leave your opinion