
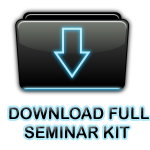
MIMO is also used in Mobile Radio Telephone standards such as recent 3GPP and 3GPP2 standards. In 3GPP, HSPA+(High Speed Packet Acess Plus) and LTE(Long Term Evolution) standards take MIMO into account. As spectral bandwidth is becoming an ever more valuable commodity for radio communications systems, techniques are needed to use the available bandwidth more effectively. MIMO wireless technology is one of these techniques. This makes MIMO wireless technology one of the most important wireless techniques to be employed in recent years.
· Functions of MIMO:
MIMO can be sub-divided into three main categories.
Precoding is multi-stream beamforming in the narrowest definition. In more general terms, it is considered to be all spatial processing that occurs at the transmitter. In (single-layer) beamforming, the same signal is emitted from each of the transmit antennas with appropriate phase (and sometimes gain) weighing such that the signal power is maximized at the receiver input. The benefits of beamforming are to increase the received signal gain, by making signals emitted from different antennas add up constructively, and to reduce the multipath fading effect. In the absence of scattering, beamforming results in a well defined directional pattern, but in typical cellular conventional beams are not a good analogy. When the receiver has multiple antennas, the transmit beamforming cannot simultaneously maximize the signal level at all of the receive antennas, and precoding with multiple streams is used.
Spatial multiplexing requires MIMO antenna configuration. In spatial multiplexing, a high rate signal is split into multiple lower rate streams and each stream is transmitted from a different transmit antenna in the same frequency channel. If these signals arrive at the receiver antenna array with sufficiently different spatial signatures, the receiver can separate these streams into (almost) parallel channels. Spatial multiplexing is a very powerful technique for increasing channel capacity at higher signal-to-noise ratios (SNR). The maximum number of spatial streams is limited by the lesser in the number of antennas at the transmitter or receiver. Spatial multiplexing can be used with or without transmit channel knowledge. Spatial multiplexing can also be used for simultaneous transmission to multiple receivers, known as space division multiple access.
Diversity coding techniques are used when there is no channel state information at the transmitter. In diversity methods, a single stream (unlike multiple streams in spatial multiplexing) is transmitted, but the signal is coded using techniques called space time coding. The signal is emitted from each of the transmit antennas with full or near orthogonal coding. Diversity coding exploits the independent fading in the multiple antenna links to enhance signal diversity. Because there is no channel knowledge, there is no beamforming or array gain from diversity coding. Spatial multiplexing can also be combined with precoding when the channel is known at the transmitter or combined with diversity coding when decoding reliability is in trade-off.
· Forms of MIMO:
The terminology and methods used in MIMO can differ from system to system, however most fall into one of two categories:
SU-MIMO (Single User - MIMO) - this utilizes MIMO technology to improve the performance towards a single user.
MU-MIMO (Multi User - MIMO) - this enables multiple users to be served through the use of spatial multiplexing techniques.
The STC (Space Time Coding) method is typically used in SU-MIMO. This utilizes multiple antennas to transmit the same information, however the data on each antenna is diversity coded, i.e. intelligently reordered in time. The following diagram illustrates this concept. In a standard SISO (Single Input Single Output) or SIMO (Single Input Multiple Output) system, only a single transmit stream is used (this is illustrated as the blue line). It can be seen that the additional diversity stream (green line) is time coded and transmitted from a second antenna, i.e. spatial diversity. This provides a combination of space and time coding. If interference / errors occur on one of the streams, the other is still able to carry the data successfully. In addition, if there was interference in the time domain, which affected both streams (denoted by the “X” in the diagram) the bits lost are duplicated at different times on the different streams. This permits the system to handle more interference and thus enables the use of HOM (Higher Order Modulation) schemes and / or reduction in FEC (Forward Error Correction) overhead. As such, STC can either provide users with a more efficient connection, or it could be used to extend the range of a cell.
Following on from the STC concept; if a device is able to differentiate between two streams carrying STC coded information, it is also true that it could differentiate between streams carrying different information. This is termed SM (Spatial Multiplexing) and can be deployed in both SU-MIMO and MU-MIMO systems. As an example, if one stream carries 20Mbps (1/1 coding, i.e. minimal error protection) in the SU-MIMO deployment, a device could receive 40Mbps. This assumes two transmit antennas are used and is typically referred to as 2x2 (2 transmit and 2 receive). In addition, if it was deployed as a 4x2 (4 transmit and 2 receive) then it could potentially handle 80Mbps. However, it should be noted that 4x2 configurations are harder to deploy and increase the signal processing required in addition to demanding a higher cost.
In comparison, spatial multiplexing could be deployed when offering MU-MIMO. As such, independent streams can be assigned to different users, hence spatially multiplexed.
In both cases, spatial multiplexing in the SU-MIMO and MU-MIMO modes provide a very powerful technique for increasing channel capacity when the SNR (Signal to Noise Ratio) is high.
To truly optimize the channel efficiency, some systems offer the ability to support AMS, or Adaptive MIMO Switching. The following diagram illustrates how a system could utilize a mixture of Spatial Multiplexing and Space Time Coding. As can be seen in the diagram, a device close to the base station could use Spatial Multiplexing, and thus increase network performance, whereas a mobile on the edge of the cell could use Space Time Coding. The latter providing better resilience to interference.
Spatial multiplexing techniques makes the receivers very complex, and therefore it is typically combined with OFDMA (orthogonal frequency division multiple access), where the problems created by multi-path channel are handled efficiently. The set of antennas used in MIMO systems based on TDMA (time-division multiple access) and OFDMA techniques can only serve one user at a time, while the set of antennas in MIMO systems using spatial division multiple access (SDMA) can serve as many different users as the number of antenna at the base station. This enable users to not use handsets with multiple antennas at receiver, as base station can multiplex users in space domain using multiple antennas at both transmitter and receiver.
· MIMO System Model And Its Applications
In MIMO systems, a transmitter sends multiple streams by multiple transmit antennas. The transmit streams go through a matrix channel which consists of all Nt Nr paths between the Nt transmit antennas at the transmitter and Nr receive antennas at the receiver. Then, the receiver gets the received signal vectors by the multiple receive antennas and decodes the received signal vectors into the original information. A MIMO system for n transmit and m receive antennas is modelled as
where and are the receive and transmit vectors, respectively, and and are the channel matrix and the noise vector, respectively.
Because multiple data streams are transmitted in parallel from different antennas there is a linear increase in throughput with every pair of antennas added to the system. An important fact to note is that unlike traditional means of increasing throughput, MIMO systems do not increase bandwidth in order to increase throughput. They simply exploit the spatial dimension by increasing the number of unique spatial paths between the transmitter and receiver. However, to ensure that the channel matrix is invertible, MIMO systems require an environment rich in multipath. A reasonable 5x5 system can provide 40x increase in capacity for the same signal to noise ratio (SNR)
MIMO can be used to advance such applications as:
I. Streaming video, music
II. Video surveillance
III. Voice over Internet Protocol (VoIP)
IV. Video conferencing
V. Interactive gaming
VI. Mobile TV
· MIMO Antenna Design:
MIMO antenna design has four fundamental principles to adhere to
• Low envelope correlation between antennas
Low envelope correlation can result from having sets of antenna patterns that are diverse in the spatial, phase and polarization senses. In other words, if all antenna patterns are looking in different directions or in different ways (phase / polarization) one has low correlation.
• Large individual antenna mean effective gain (MEG)
MEG refers to the gain of an antenna in a particular environment relative to some reference antenna (e.g. isotropic radiator). In other words, it is a measure of how well an antenna receives power for a particular scattering environment.
• All antennas with roughly the same mean effective gain
The MEG of each antenna needs to be roughly the same for a balanced system
• Low total array reflection coefficient (TARC)
Antennas radiate simultaneously and must have minimal crosstalk pairs.
The biggest challenges in MIMO antenna design are as follows
• There are simple design rules to follow for an ideal scattering environment, but deviation from the ideal case leads to antenna design with no design guidelines.
• Low envelope correlation is at odds with large mean effective gain. If the region we wish to have large gain constitutes a small window of the far-field, it means we must have diversity in this window while simultaneously having large gain in said window – both of which are clearly at odds.
• There is a strong push towards mobile communications and MIMO on handhelds, which leads to closely spaced radiating elements, thereby conflicting with all requirements of MIMO antenna design.
A reconfigurable microstrip dipole antenna is a solution for Multiple Input Multiple Output (MIMO) communication systems making use of Orthogonal Frequency Division Multiplexing (OFDM). When applied to closely spaced antennas, this method can increase link capacity. The benefits of this novel antenna solution can be demonstrated by channel capacity measurements taken in an indoor environment with a 2 by 2 MIMO system.
Reconfigurable antennas have been used to achieve pattern and frequency diversity in Single Input Single Output (SISO) links , and recently they have been suggested for use in MIMO systems. The reconfigurable antenna array consisted of two microstrip dipoles with variable electrical length separated by a quarter wavelength. The two active elements of the array could be reconfigured in length using PIN diode switches. The setting of the switches resulted in different geometries of the antenna and, consequently, different levels of inter-element mutual coupling and array far-field radiation patterns. This variable pattern diversity allowed multipath signal components to and from each antenna to be weighted differently depending upon the configuration of the antennas used. The overall goal of a system making use of this reconfigurable antenna technology would be to choose the configuration of switches in an environment/channel adaptive fashion to decrease MIMO spatial channel correlation and subsequently maximize link capacity.
The antenna solution was realized using an array of two microstrip dipoles illustrated in Fig.. The length of the dipole-arm strip, and subsequently, the geometry of the antenna, could be changed using two PIN diode switches (MA4P789). Using this technique, it was possible to define two configurations for the antenna: one in which both the switches are turned on (“long” configuration) and another in which they are turned off (“short”configuration). Both configurations were shown to efficiently radiate and be matched at a frequency of 2.45 GHz. In particular the “short” configuration (half wavelength microstrip dipole) performed better than the “long” configuration (three quarter wavelength microstrip dipole) both in radiation efficiency n (“long” configuration n=62%, “short” configuration n=75%) and in matching condition. Different radiation patterns for each possible combination of antenna configurations were generated by placing two of these reconfigurable antennas a quarter wavelength apart. It was then possible to define four configurations of the two element antenna array (both antennas “long”, both antennas “short”, one antenna “short” and the other “long” and vice versa) at each side (transmitter and receiver) of the MIMO-OFDM communication link. The proper choice of the receiver and transmitter array switch configurations was the one which decreased MIMO spatial channel correlation and maximized channel capacity for a particular multipath environment. For example, the optimal choice could be determined by switching through all the possible length configurations at transmitter and receiver whenever the capacity experienced by the link fell below a predefined threshold. Current research is focused on: i.) techniques for integrating a switch configuration selection process into practical MIMO-aware ad hoc network nodes and ii.) determining the interval of time over which a switch configuration is optimal before a new configuration needs to be selected
These antenna configurations were tested for 2 by 2 MIMO with keeping transmitter stationary, while the receiver was moved between several different locations. The channel matrix was measured for five different positions of the receiver and for all sixteen possible configurations of the antenna system (4 configurations at the transmitter times 4 configurations at the receiver). The results showed that antenna solution achieved a 10% improvement in capacity for a SNR of 10 dB.
· Use Of MIMO In 3G Cellular Systems:
The growing demand of multimedia services and the growth of Internet related contents lead to increasing interest to high speed communications. The requirement for wide bandwidth and flexibility imposes the use of efficient transmission methods that would fit to the characteristics of wideband channels especially in wireless environment where the channel is very challenging. In wireless environment the signal is propagating from the transmitter to the receiver along number of different paths, collectively referred as multipath. While propagating the signal power drops of due to three effects: path loss, macroscopic fading and microscopic fading. Fading of the signal can be mitigated by different diversity techniques. To obtain diversity, the signal is transmitted through multiple (ideally) independent fading paths e.g. in time, frequency or space and combined constructively at the receiver. Multiple input- multiple-output (MIMO) exploits spatial diversity by having several transmit and receive antennas.
Despite all the excitement surrounding MIMO’s promised capacity increases, it is quite a different matter to apply it successfully to a commercial cellular system. Nevertheless, MIMO’s enormous data rate incentives have motivated much interest from 3G cellular providers and equipment manufacturers, and MIMO is being widely considered for cdma2000 (3GPP2) and WCDMA (3GPP), particularly for the high-data rate modes such as EV-DV, EV-DO, and HSDPA. All well-designed cellular systems are by nature interference-limited: if they were not, it would be possible to increase the spectral efficiency by lowering the frequency reuse or increasing the average loading per cell. In the downlink of a cellular system, where MIMO is expected to be the most profitable and viable, there will be an effective number of NMt interfering signals, if the number of non-negligible neighboring base stations is N.
The OCI Problem in Cellular MIMO Communications
There have been a few notable preliminary studies on interference-limited MIMO networks with the general conclusion that other-cell interference severely degrades the overall capacity of spatial multiplexing MIMO systems. MIMO receivers are able to decode the parallel data streams by suppressing the spatial interference between the signals sent from the Mt adjacent transmit antennas. The root cause of the degradation is that the MIMO receiver has to focus on suppressing the spatial interference introduced by the multiantenna transmitter; consequently, there are not sufficient degrees of freedom available to also suppress the co-channel interference, be it from other users in the same cell or from other cells (other-cell interference).Recently, a closed-form expression for interference-limited MIMO cellular system performance was developed, specifically for the outage probability and outage capacity of a MIMO-CDMA system with linear receivers. Although one of the primary advantages of CDMA is its resilience to co-channel interference, OCI can severely degrade the overall capacity of spatial multiplexing even in CDMA systems. In particular, it can be seen that a conventional 1 * 1 cellular CDMA system in fact performs similarly to an 8 * 8 system with the same total rate (and hence 8 times the spreading factor). Although a suboptimal Minimum Mean Square Error (MMSE) receiver can reduce the noise enhancement by allowing some residual spatial interference, the overall improvement is incremental. While the problem of other-cell interference has existed in cellular systems for many years, its effect on MIMO systems is far more severe, and also more intriguing. Traditional OCI-reduction techniques have been quite limited, such as sectoring cells using simple directional antennas, or increasing the frequency reuse distance.
OCI mitigation techniques
In this section, we outline possible OCI mitigation techniques in 3G cellular MIMO systems and discuss their feasibility.
A. Frequency reuse
Traditionally, frequency reuse has been adopted for OCI reduction in cellular systems owing to its simplicity and practicality. Frequency reuse effectively reduces OCI by spacing the competing transmissions farther away, and particularly benefits users near the cell boundaries. This of course reduces the spectral efficiency, since now only 1=f of the available spectrum is used each cell, if f is the “frequency reuse factor”, which is generally between 3-7 in non-CDMA systems. One of the great capacity and deployment advantages of CDMA is its allowance of universal frequency reuse (i.e. f = 1), so simple static frequency reuse is not an attractive option for MIMO-CDMA in 3G cellular. This will be shown more concretely in the next section.
B. Advanced Receiver Techniques
Interference-aware MIMO receivers can significantly attenuate the OCI and hence greatly improve system performance. Note here that we are focused on suppressing other-cell interference, as opposed to self-cell interference. The latter is usually the emphasis in most multiuser receiver literature. However, cancelling the self-cell interference is not that interesting in 3G CDMA since orthogonal codes are used in the downlink, so a chip-level equalizer is the most effective means for reducing self-cell interference, since minus multipath, the self-cell users do not interfere with each other. Such chip-level equalizers are under wide investigation in industry, and a 3GPP study group has been initiated by Nokia for this very purpose. So instead, we overview advanced signal processing options available to the receiver to suppress other-cell interference, and explain the key challenges these techniques face if they are to contribute to the adoption of MIMO in 3G cellular systems.
· (Near) Maximum Likelihood Multiuser Detection:
If instantaneous information on the channels of interferers is available, maximal likelihood (ML) Multiuser Detection (MUD) is known to minimize the bit error probability in a multicellular MIMO system. However, not only is this instantaneous channel information difficult to attain for neighboring base stations, the complexity of such a receiver is prohibitive for a low-power mobile unit. For an Mr £Mr MIMO system using M-QAM , the complexity is on the order of MNMr , where N is the number of interferers . Even for simple cases this is well beyond reasonable implementation complexity. An alternative is the sphere decoder, which searches for the optimal solution in a sphere around an estimate of the received codeword. Sphere decoders can deal with moderate NMr but still fail for large numbers of antennas or interferers. Additionally, they have variable complexity making implementation difficult. VLSI implementations are under development but their viability in power hungry mobiles is still open to debate
· The MMSE Receiver:
Due to the complexity problems associated with the ML receiver, a natural approach is to consider a linear approximation to the ML receiver. Here, two classes of MMSE receivers can be considered: one that knows the interferer’s channels (MMSE MUD) and one that does not know anything about the interference other than its autcorrelation (OCI-blind MMSE). Naturally, the MMSE MUD technique has superior performance, but suffers from two important problems. First, as in the ML detector, a major difficulty is attaining instantaneous channel knowledge for the interfering base stations. Secondly, because Mt antennas at each of N interfering base stations must be treated as Mt independent interferers (for a total of MtN interferers), effectively suppressing all of this interference is very difficult with only Mr ¿ MtN receive antennas. One simplification, which is being looked at by Cingular and others, is to cancel just the pilot and common channel signals of the strongest interfering base station, which also alleviates the channel estimation requirements. However, the network-wide capacity gains from doing this are predicted to be around 10-25%, which is perhaps worthy of implementation but not a major leap forward. Due to these challenges, an OCI-blind MMSE receiver can be implemented with only a power estimate of the total received signal Ryy instead of exact channel knowledge for all the interfering signals.
· Interference Cancelling Receivers:
In single antenna systems, nonlinear receivers often provide a desirable tradeoff between performance and complexity , relative to ML receivers at one extreme and linear receivers at the other. In multicell MIMO systems, group detection techniques have a natural appeal, in which information bits for each “group” (cell) are detected sequentially . One of the most popular among various group detection techniques is group decision feedback MUD, an extension from BLAST. This receiver detects one MIMO system at a time and then feeds the tentative decisions to other group detectors for interference cancellation. Although successive interference cancellation is asymptotically optimal under the assumption of perfect interference cancellation, it is very susceptible to inaccurate channel estimates, and also is currently deemed too complex for low-power mobile units.
An important shortcoming of the above techniques is that most require channel information for the neighboring base stations, which is traditionally not available. In 3G cellular systems such as HSDPA and EVDV, mobile stations periodically monitor pilot channels of neighboring base stations to assist with handoff. These pilot signals could also be used to gather the required channel knowledge for the above multiuser receivers. For 3G CDMA systems, a parallel searcher structure might help to acquire these signals but it would necessarily increase the complexity and the power consumption of the mobile stations.
C. Advanced Transmitter Techniques
For many systems, in both theory and practice, transmitter adaptation has been shown to be a highly profitable means of exploiting channel conditions. A well-known example is adaptive modulation, which is used in EV-DO and HDSPA to achieve high data rates for users with good channels. Another method is the closed-loop diversity mode of WCDMA, which approximates the maximum ratio transmission beamforming vector. Transmitter-based techniques have the additional merit in a cellular downlink of transferring the complexity burden from the mobile unit to the base station, where higher complexity is more tolerable. In this section, we consider methods of using channel knowledge at the transmitter to help suppress other-cell interference using advanced signal processing techniques.
1) Cooperative Encoding:
If the received interference signals are known to the transmitters,cooperative encoding among neighboring base stations can suppress other-cell interference. Such a joint encoding scheme is an example of so-called dirty paper coding (DPC), which has been shown to achieve the maximum capacity of the multiuser MIMO downlink channel . However, joint encoding is nearly impossible to achieve in practical systems because it requires precise time and phase synchronization of the signals transmitted from multiple base stations, and exact channel knowledge at all the transmitters. Even though the 3G cellular systems have base station controllers (BSC) or radio network controllers (RNC), which control multiple base stations , the precise accuracy required for DPC renders this technique as a theoretical upper bound, rather than a practical solution.
2) Closed-Loop MIMO Diversity Schemes:
Given Mt > Mr antennas, it is possible to improve the performance of spatial multiplexing by using channel state information at the transmitter.One example is antenna subset selection where the best Mr of Mt antennas are selected. Other examples include eigen beamforming and transmit precoding. These schemes require knowledge of channel state information at the transmitter; efficient limited feedback strategies for this purpose have already been developed . Closed-loop diversity schemes provide a diversity improvement of Mt - Mr + 1 and additional array gain, which reduces the required transmit power for spatial multiplexing, and hence the interference caused to neighboring cells. On a system-wide level, such approaches have about the same impact on OCI as transmit diversity.
3) MIMO Combined with Transmit Beamforming:
While the name “beamforming” has been applied to a large number of diverse techniques, the common idea is to use signal processing at the transmitter to maximize the signal energy sent to the desired user, while minimizing the interference sent towards interfering users. Some of these techniques include Space Division Multiple Access (SDMA), eigenmode beamforming for high capacity, and maximal ratio transmission for diversity . Beamforming can be combined with spatial multiplexing to give multiple high data rate streams, although the dimensions used for beamforming do reduce the number of simultaneous data streams that can be transmitted. Although beamforming is traditionally envisioned to battle self-cell interference since it requires the complete interference statistics for each user at the transmitter, in doing so it reduces the other-cell interference. Despite its technical merits, beamforming has not found commercial adoption due to its requirement for rich channel knowledge at the transmitter.
Strategic Approaches To The OCI Problem
As discussed in the prior section , the numerous recently proposed methods for mitigating OCI in cellular MIMO systems have some important shortcomings when viewed in a practical context such as a 3G cellular system. Although some of these techniques have important merits and are being actively researched and considered, it is important to consider strategic approaches to handling OCI in cellular systems that do not require realtime information about the other-cell interference. In this section, we propose two system-level techniques that can in principle be combined with the previously discussed advanced signal processing algorithms to yield even larger gains, if and when these become viable. While these two techniques will effectively reduce OCI and increase spectral efficiency for any interference limited cellular system, the gains are particularly impressive for multicell MIMO-CDMA due to its severe degradation in the face of OCI.
A. Cooperatively Scheduled Transmission
Recent work by the authors and others has investigated the possibility for neighboring base stations to cooperatively schedule their transmission, a generalization of the concept of spatial frequency reuse. This scheduling could either be dynamic (and hence require simple intercell coordination) or pre-determined based on a universally shared time-hopping sequence. Just as frequency reuse achieves OCI reduction at the expense of decreasing spectral efficiency by the frequency reuse factor, cooperatively scheduled transmission reduces OCI at the expense of decreasing throughput by the transmit duty cycle. There are two important advantages of cooperatively scheduled transmission relative to traditional frequency reuse. First, universal frequency reuse can still be adopted, achieving an interference-averaging effect, simplifying frequency planning in deployment, and reducing the number of required frequency channels for the system. Second, cooperatively scheduled transmission achieves an additional gain termed expanded multiuser diversity if straightforward opportunistic scheduling is employed among neighboring base stations.
For a 2x2 MIMO system with cooperatively scheduled transmission, the capacity gain of cooperatively scheduled transmission over a traditional frequency reuse system (f = 7) is shown in Figure , where all the users in each cell are assumed to be uniformly distributed. As it is expected, cooperatively scheduled transmission exploits expanded multiuser diversity and achieves higher capacity than traditional frequency reuse (f = 7). The expanded multiuser diversity gain in terms of capacity is about 1.5 bps/Hz. It should be stressed however that the main motivation for introducing cooperatively scheduled transmission is not to increase the overall capacity relative to universal time and frequency reuse, but to support the many users that are near cell boundaries, so they can achieve acceptable data rates.
B. Distributed antenna architecture
Another strategic approach to reducing the other-cell interference problem is to adopt a distributed antenna architecture, which has been considered in the past as an effective means of extending coverage, eliminating dead spots, and lowering the blocking probability. In such an architecture, antenna modules are geographically distributed throughout the original cell to reduce the access distance for each node, and is then connected to a home base station (or central unit) via dedicated wires, fiber optics, or an exclusive RF link. An example of the distributed antenna cellular structure is given in Figure
.
Despite the increased equipment costs associated with this architecture, simple examples are already being widely deployed in 3G cellular systems by equipment manufacturers and service providers such as Korea Telecom, ADC, SK Telecom, and Cingular, due to the aforementioned qualities and also the ease of installation and maintenance. Since a distributed antenna architecture lowers the aggregate transmit power, it is natural that such a scheme will lower the amount of interference caused to neighboring cells. By transmitting to each user from the antenna closest to it (or more precisely, with the best channel), a form of macroscopic spatial diversity is also introduced to the system, with the net result being a much higher average received SINR, with particularly large gains for users near the distributed antennas (and hence the cell edges). The large SINR gain achieved from deploying distributed antennas could be the key to the success of large-scale MIMO cellular systems with universal frequency reuse.
THE FUTURE OF MIMO IN 3G CELLULAR
The demand for mobile data services has increased by an average of 160% in the year 2009 alone, and some mobile carriers have experienced even more aggressive growth numbers. According to a recent forecast, the global mobile data traffic is expected to continue to double every year through 2014, leading to a global compound annual growth rate of 108% . To meet these needs, advanced features are investigated for inclusion in future releases of 4G standards (WiMAX evolution and LTE Advanced).Within the range of system development, LTE-Advanced and WiMAX 2 can use up to 8x8 MIMO and new reference signals have been introduced to support both demodulation and channel state information estimation. Hence, special attention has been given to the signaling needed for more advanced SU/MU-MIMO schemes. However, one of the key challenges facing the deployment of MIMO technology in cellular networks is the sensitivity of MIMO receivers to interference. Since cellular systems are inherently interference-limited, this introduces a fundamental conflict. On one hand, system designs should minimize transmit power and rate in order to reduce the interference caused to neighboring cells. On the other hand, MIMO systems by nature increases the amount of data transmitted and hence requires a larger received SINR. Recent research approaches to this difficult problem have focused on advanced signal processing techniques at the receiver and transmitter as a means of reducing or cancelling the perceived interference.
Allowing for some simple back-channel communication among neighboring base stations, as is typically the case due to the need to coordinate handoffs and other operations, cooperatively scheduled transmission can help reduce the interference and increase the net spectral efficiency. Even larger gains are possible with a distributed antenna architecture, which is already being widely considered as a means to cheaply extend coverage and furnish additional capacity. Distributed antennas allow the transmit power to be dramatically lowered, which increases the average net received SINR by over an order of magnitude. While this improved SINR will benefit the capacity of any cellular network, the gain will be particularly large in a MIMO system due to its sensitivity to interference.
Conclusion:
MIMO system is nothing but the use of multiple antennas at both transmitter and receiver. This is used to improve the link reliability and data throughput without additional bandwidth and transmit power. Multi-user MIMO and single-user MIMO are the two forms of MIMO with functions such as precoding, diversity coding and special multiplexing. Reconfigurable antennas have been used to achieve pattern and frequency diversity in MIMO. This article has documented most of these techniques suffer from important practical shortcomings in terms of complexity and required channel information that make their successful application to 3G cellular systems. This article has proposed two simpler network-level approaches that require very little channel knowledge and effectively reduce other-cell interference through macro-diversity.
· References
Ø en.wikipedia.org/wiki/MIMO
Ø www.scribd.com
Ø MIMO in 3G Cellular Systems: Challenges and Future Directions
Jeffrey G. Andrews, Wan Choi, and Robert W. Heath Jr.April 2005
not in proper way ,shpuld be in a mannor.
ReplyDelete