
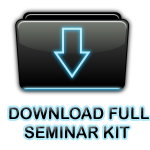
The body's defense system - called the immune system - consists of a network of specialized cells and tissues that fight infection and disease. Therapies that use the immune system to fight or prevent cancer are called biological therapies.
Vaccine development is one of the most promising and exciting fields in cancer research; numerous approaches are being studied to developed effective cancer vaccines. The aim of this form of therapy is to teach the patient's immune system to recognize the antigens expressed in tumor cells, but not in normal tissue, to be able to destroy these abnormal cells leaving the normal cells intact. In other words, is an attempt to teach the immune system to recognize antigens that escaped the immunologic surveillance and are ‘tolerated’ by it, therefore able to survive and, in time, disseminate. However each research group developing a cancer vaccine, uses a different technology, targeting different antigens, combining different carriers and adjuvants, and using different immunization schedules.
Unlike the vaccine you might receive for the flu, most cancer vaccines aren't intended to prevent you from getting cancer. Rather, the majority of cancer vaccines being studied aim to condition your body to recognize cancer cells as invaders. Vaccines may be used to attack cancer cells in several different ways. In one method, the vaccine consists of cancer cells that are inactivated, usually through radiation, and injected into your body. These cancer cells can't grow and form new cancer, but they still carry the signals that activate your immune system to attack cancer in your body. Some vaccines are made of cells from your own cancer. Still others are made of cells from your immune system (dendritic cells). Scientists manipulate these cells to recognize cancer cells and then inject the manipulated cells into your body. Once in your body, the manipulated cells target the cancer cells, as well as recruit other cells in your immune system to join the fight.
A vaccine is a substance designed to stimulate the immune system to launch an immune response. This response is directed against specific targets, or antigens, that are part of the vaccine. An antigen is any substance that the immune system recognizes as foreign.
Vaccines can be made using specific types of molecules from viruses or cells, including molecules from bacterial cells or human cells. These molecules may contain a single antigen or several different antigens. Carbohydrates (sugars), proteins, and peptides (pieces of proteins) are among the types of molecules that have been used to make vaccines. Molecules of DNA or RNA that contain genetic instructions for one or more antigens can also be used as vaccines.
In addition, whole viruses or cells, or parts of viruses or cells that contain different types of molecules, can be used to make vaccines. The flu vaccine, for example, is made using inactive whole flu viruses. If whole human cells are used as vaccines, they are usually treated with enough radiation to keep them from dividing (growing and multiplying) or enough to kill them.
It has been more than 100 years since the first reported attempts to activate a patient's immune system to eradicate developing
cancers. Although a few of the subsequent vaccine studies demonstrated clinically significant treatment effects, active immunotherapy has not yet become an established cancer treatment modality. Two recent advances have allowed the design of more specific cancer vaccine approaches: improved molecular biology techniques and a greater understanding of the mechanisms involved in the activation of T cells.
These advances have resulted in improved systemic antitumor immune responses in animal models. Because most tumor antigens recognized by T cells are still not known, the tumor cell itself is the best source of immunizing antigens.
For this reason, most vaccine approaches currently being tested in the clinics use whole cancer cells that have been genetically modified to express genes that are now known to be critical mediators of immune system activation. In the future, the molecular definition of tumor-specific antigens that are recognized by activated T cells will allow the development of targeted antigen-specific vaccines for the treatment of patients with cancer.
The immune system and cancer 19
Researchers used to think that the immune system prevented cancer from growing and spreading by constantly looking to see if cancer cells are present and killing them once they are found. It was thought that the growth and spread of cancer resulted from a breakdown of the immune system. In a broken-down immune system, effective anti-cancer immune responses could not occur.
However, this theory of immune system control over cancer growth has now been shown to be only partially correct. Researchers now know that strong immune responses against cancer cells are hard to generate, and they are studying ways to strengthen the ability of the immune system to fight cancer.
Part of the problem is that the immune system has the job of knowing the difference between normal cells and cancer cells. To keep us healthy, the immune system must be able to ignore or “tolerate” normal cells and recognize and attack abnormal ones.
To the immune system, cancer cells differ from normal cells in very small, subtle ways. Therefore, the immune system largely tolerates cancer cells rather than attacking them. Although tolerance is essential to keep the immune system from attacking normal cells, tolerance of cancer cells is a problem. Therapeutic cancer vaccines must not only provoke an immune response but stimulate the immune system strongly enough to overcome its usual tolerance of cancer cells.
Another reason cancer cells may not stimulate a strong immune response is that they have developed ways to evade the immune system. Scientists now understand some of the ways in which cancer cells do this. For example, they may shed certain types of molecules that inhibit the ability of the body to attack cancer cells. As a result, cancers become less "visible" to the immune system.
Researchers are now using these advances in knowledge in their efforts to design more effective cancer vaccines. They have developed several strategies for stimulating immune responses against cancers, including the following:
v Identify unusual or unique cancer-related molecules that are rarely present on normal cells and use these so-called “tumor antigens” as vaccines.
v Intervene to make tumor antigens more visible to the immune system. This can be done in several ways:
§ Alter the structure of a tumor antigen slightly (that is, make it look more foreign) and give the altered antigen as a vaccine. One way to alter an antigen is modify the gene needed to make it. This can be done in the laboratory.
· Put the gene for a tumor antigen into a viral vector (a harmless virus) and use the virus as a vehicle to deliver the gene to cancer cells or to normal cells. Cells infected with the viral vector will make much more tumor antigen than uninfected cancer cells and may be more visible to the immune system. Cells can also be infected with the viral vector in the laboratory and then given to patients as a vaccine. In addition, patients can be infected (that is, vaccinated) with the viral vector as another way to get virus-infected cells inside the body.
§ Put genes for other molecules that normally help stimulate the immune system into a viral vector along with a tumor antigen gene.
v Use “primed” dendritic cells or other APCs as a vaccine. There are three ways to prime a dendritic cell.
§ APCs can be fed tumor antigens in the laboratory and then injected into a patient. The injected cells are primed to activate T cells.
§ Alternatively, APCs can be infected with a viral vector that contains the gene for a tumor antigen.
§ A third way to make primed APCs is to feed the cells DNA or RNA that contains genetic instructions for the antigen. The APCs will then make the tumor antigen and present it on their surface.
v Use antibodies that have antigen-binding sites that mimic, or look like, a tumor antigen. These antibodies are called anti-idiotype antibodies. They can stimulate B cells to make to make antibodies against tumor antigens. Anti-idiotype antibodies present tumor antigens in a different way to the immune system.
The classical concept of the vaccine derives from the practice of immunizing against infectious agents to prevent disease by generating a humoral immunity. Individuals are immunized against viral or bacterial antigens before they encounter the pathogenic organisms. This strategy became rapidly successful
for viruses because viral genes are relatively simple, possessing a limited number of defined antigens. However, in the case of most tumors, there is an unlimited number of potential antigens that can be the target of an immune response. In addition, it is likely that many of these antigens arise during or as a result of the tumorigenesis process. Therefore, when we talk about tumor vaccines, the most common clinical setting is one in which the induction of a systemic immune response by the vaccine occurs subsequent to, rather than before, the antigen insult. Finally, in contrast to prophylactic vaccines, the majority of cancer vaccines aim to induce a cellular, antigen-specific T-cell response.
Cancer vaccines are intended either to treat existing cancer or to prevent the development of cancer. Cancer treatment vaccines are designed to strengthen the body's natural defenses against a cancer that has already developed. These vaccines may stop an existing tumor from growing, stop a tumor from coming back after it has been treated, or eliminate cancer cells not killed by previous treatments.
Cancer treatment vaccines can be made using a patient’s own tumor antigens or cells, or someone else’s. Most tumors of a given type share many antigens. When a patient’s own tumor antigens or cells are used, the vaccine is called an autologous vaccine. When someone else’s tumor antigens or cells are used, the vaccine is called an allogeneic vaccine.
Cancer preventive vaccines are given to healthy people and are designed to target infectious agents that can cause cancer. The hepatitis B virus vaccine is an example of a cancer preventive vaccine. It is used to help prevent liver cancer. Another preventive vaccine that is being studied in clinical trials is a vaccine against human papilloma virus (HPV), which is believed to cause most cases of cervical cancer.
Cancer vaccines often have added ingredients, called adjuvants that help boost the immune response. These substances may also be given separately to increase a vaccine’s effectiveness. Many different kinds of substances have been used as adjuvants, including cytokines, proteins, bacteria, viruses, and certain chemicals.
The first reported cancer vaccine was tested over a century ago by William Coley, a New York surgeon, who used extracts of pyogenic bacteria to stimulate antitumor immune responses. Numerous subsequent clinical trials have been conducted, particularly using irradiated whole tumor cells mixed with bacterial adjuvants such as Calmette-Guérin bacillus or Corynebacterium parvum. Studies of this approach for patients with malignant melanoma and renal cell and colorectal carcinomas have demonstrated small but significant clinical effects. These early studies established a basis for using immune modulators in a paracrine fashion to generate antitumor immunity.
How are cancer vaccines different from vaccines that prevent infections? 7
Therapeutic cancer vaccines are not designed to prevent cancer. Instead, they are designed to spur the immune system into recognizing tumor cells as foreign invaders so that they may be destroyed by the host immune system. Tumor cells often express distinct antigens known as tumor-associated antigens (TAAs). One of the greatest problems with developing cancer vaccines has been that most TAAs are also present in normal cells. Because the immune system sees these antigens as self-antigens, no immune response is mounted. If the immune system can be taught to recognize the TAAs as foreign, an immune response can be mounted against the tumor. Several TAAs have been identified that are found in specific types of cancers, but not in normal cells. By targeting these TAAs with cancer vaccines, cancer vaccines can induce the immune system to attack cancer cells while leaving normal, healthy cells largely intact. Currently, cancer vaccines targeting cancers of the breast, prostate, liver, kidney, pancreas, and lung, as well as melanoma and certain types of leukemias and lymphomas are in clinical trials.
Cancer vaccines can be broadly classified into two categories: those that target shared antigens and those that target unique antigens.
Shared-antigen cancer vaccines
Shared antigens are antigens that exist in normal tissues but are overexpressed in cancer cells. Because they are expressed in normal tissues, there is an increased tolerance for them, making shared antigens less likely to stimulate immune response. Moreover, if a cancer vaccine does overcome tolerance, autoimmunity can often be an unwelcome side effect.
The majority of therapeutic cancer vaccines in development use a shared-antigen approach and differ only in the particular antigens used or the method used to attempt to overcome tolerance. Clinical testing of shared antigens to date has been largely confined to a small number of cancers, and the number of shared antigens necessary to target every cancer cell in any one patient and achieve clinical benefit across diverse patient populations is unknown.
Unique-antigen cancer vaccines
Unique antigens are antigens that occur only in cancer cells and do not exist in normal tissues. They arise from mutations that occur as the tumor cells grow uncontrollably. The vast majority of unique antigens are not only specific to cancer cells but also specific to each individual patient. As a result, the unique-antigen approach is potentially applicable to all cancer types, from solid tumors to hematological malignancies.
Currently, the only way to produce a cancer vaccine using these unique antigens is by employing an autologous approach — meaning that the antigens are derived from the same individual they are used to treat. Preliminary indications of clinical benefit associated with autologous vaccine treatment have been observed in every indication tested — close to a dozen types of cancer. Autologous cancer vaccines in development include those utilizing whole cells, tumor lysates, RNA or heat shock proteins.
Whole cells/lysates/RNA
Early attempts at autologous cancer vaccines involved whole cell preparations or lysates of a patient’s tumor, which were shown to be unsuccessful, as unpurified cellular contents send both stimulatory and suppressive immune signals.
Although some success has been achieved using newer approaches, the complexity of the manufacturing process, issues with sterility, and difficulty in characterization of these complex autologous products make them less attractive from a commercial and regulatory standpoint.
Heat shock proteins
Antigenics’ approach to cancer vaccines is based on heat shock proteins (HSPs), a ubiquitous family of proteins believed to play a role in the presentation of antigens on the cell surface to help the immune system recognize diseased cells. Among cancer vaccine approaches — autologous or otherwise — the HSP approach has achieved the highest degree of independent validation by researchers worldwide, and has shown indications of clinical benefit in seven cancer types to date.
The company’s HSP-based cancer vaccines Oncophage and AG-858 are designed to utilize the entire repertoire of unique antigens from an individual patient’s tumor to stimulate a strong tumor-specific immune response. Both vaccines are sterile protein preparations, manufactured using a simple and cost-effective
T-CELL & TUMOR - IMMUNE RESPONSE 8-10
Role of T-cells in the antitumor immune response
Several preclinical studies have demonstrated that activation of both CD4+ and CD8+ T cells is critical for generating the most potent antitumor immune responses. These antigen-specific T-cell responses are initiated by professional antigen-presenting cells (APCs) . Any protein in the tumor cell is a potential tumor antigen. These antigens are released by secretion, shedding, or tumor lysis and captured by APCs. Uptaken antigens are processed and presented by major histocompatibility complex (MHC) class I and MHC class II molecules for priming and activation of CD8+ and CD4+ T cells, respectively
Eight– to 10–amino acid peptide fragments are presented on MHC class I molecules, whereas peptides presented on class II molecules are between 12 and 20 amino acids long. Activated CD4+ T cells provide important costimulation via cytokine secretion, which can initiate and also amplify the CD8+ T-cell response. In addition, memory CD4+ T cells play a critical role in maintaining the protective immunity. Ultimately, activated antigen-specific CD8+ T cells become cytotoxic and lyse tumor cells.
If T cells have the ability to recognize and lyse tumors, then why doesn't the immune system naturally eradicate developing cancers? This question has perplexed tumor immunologists for years. It is now becoming clear that when the immune system encounters a new antigen, which in the case of tumor antigens might have arisen during the tumorigenesis process, the outcome may be tolerance rather than activation.
The context in which the antigen is presented to the immune system seems to determine whether or not a T cell becomes activated. In the absence of appropriate costimulatory signals, engagement of the T-cell receptor itself can lead to ignorance, anergy, or even apoptotic death of the T cell , scenarios that are the complete opposite of what one might wish for tumor antigen-specific T cells. There are many examples of these mechanisms of tolerance in animal tumor models.
To overcome these mechanisms of tolerance, different vaccine approaches have been developed that use tumor cells (tumor cells provide the antigen source because most tumor antigens are still not known) that have been genetically modified to either express the critical surface costimulatory molecules (such as B7 and intercellular adhesion molecule) required for direct T-cell activation or express costimulatory cytokines that attract and activate bone marrow–derived professional APCs, which can themselves effectively process and present tumor antigens as well as constitutively express the appropriate surface costimulatory molecules required for T-cell activation .
There are successful examples of both approaches in preclinical models, and both strategies are currently being tested in clinical trials . However, the recent advances in our understanding of the mechanism of T-cell activation strongly favor exploiting professional APCs because this strategy may be the most natural way of activating potent and long-lasting antitumor immunity.
In animal models, it has been shown that bone marrow–derived APCs are indeed able to endocytose tumor antigens and present them not only to CD4+ T cells but also to CD8+ T cells, a process called "cross-priming". There is also evidence from in vitro experiments that dendritic cells efficiently present antigen derived from apoptotic cells stimulating class I–restricted CD8+ cytotoxic T cells (CTLs). The ability to target antigens to the MHC class I and class II pathway by a single APC, thereby presenting antigenic epitopes to both CD4+ and CD8+ T cells, seems to facilitate the provision of CD4+ T-cell "help" to CD8+ T cells. This occurs via CD4+ T cell–derived lymphokines and also through the APC itself via the interaction of CD40 ligand on T cells and CD40 on APCs..
The cross-priming mechanism suggests that the vaccinating tumor cells do not need to be HLA compatible with the host to generate specific antitumor immunity, thereby providing rationale for a more generalized and feasible whole-cell tumor vaccine approach. Two recent reports using murine cancer models, one evaluating an allogeneic tumor vaccine in which the antigen is not known and one evaluating an allogeneic tumor vaccine that expresses the human papilloma virus (HPV) antigen E7, support the feasibility of an allogeneic vaccine approach. Clinical trials have recently been initiated to test cytokine-secreting allogeneic vaccines in patients with various cancers.
Tumor antigens recognized by T-cells
Techniques are now available to molecularly define tumor-specific antigens that serve as T-cell targets . As a result, the field of tumor antigen identification has exploded. Eventually, the entire spectrum of tumor-specific antigens capable of being recognized by T cells should be identified. Identification of these tumor antigens that are T-cell targets provides the basis for antigen-specific immunotherapy.
Two strategies, a genetic approach and a biochemical approach, are currently used to identify MHC class I-restricted tumor antigens. Both of these methods require the availability of tumor-specific MHC class I–restricted T-cell lines or clones to provide a readout of T-cell recognition of antigen (which can be either the measurement of target-cell lysis or cytokine release).
Unlike strategies that search for evidence of immune responses to candidate antigens, such as the products of mutated oncogenes or tumor suppressor genes, these methods do not limit the search, but rather attempt to define the actual antigen recognized by CD8+ T cells obtained from patients. The genetic approach has been successful in identifying two murine tumor antigens and over 10 human melanoma antigens.The biochemical approach has been successfully adapted to identify two murine tumor antigens and one human melanoma antigen. Recently, a third approach for identifying CTL target antigens, which uses the serum from patients with cancer to screen cDNA libraries generated from tumor cells
, has been described. This method has already identified two melanoma antigens (melanoma antigen 1 [MAGE-1] and tyrosinase), both originally identified by cloning the epitopes recognized by CTL, as well as a novel human esophageal cancer–associated antigen (NY-ESO-1). Identification of T-cell antigens with this method depends on high-titer antitumor immunoglobulin G antibody in the patient's serum. The development of such high-titer antibodies, however, requires the help of antigen-specific CD4+ T cells. In addition, a classical biochemical approach for protein purification has led to the identification of one murine MHC class II antigen.
Table 1. Potential Sources of Tumor Antigens.
|
Table 1 lists the categories of antigens that are potential in vivo tumor-associated antigens recognized by T cells. Examples of antigens in each category have already been identified. Malignant melanoma-associated antigens form the largest database of identified human tumor antigens recognized by T cells. So far, melanoma antigens fall into three of these categories. Currently, the largest group are the tissue-specific differentiation antigens. The identification of differentiation antigens, antigens that are present on normal melanocytes as well as on melanoma cells, was unexpected, because it was previously thought that T cells specific for a self-antigen would be deleted or at least functionally tolerized. The first differentiation antigen that was identified was tyrosinase, a central enzyme of melanin synthesis. Others have subsequently been identified, including MART-1/Melan A, gp100, TRP-1, and TRP-2. These tissue-specific antigens are shared common antigens overexpressed by about 50% of malignant melanomas tested, making them excellent targets of immunization. Many of the identified antigenic peptides that derive from these proteins are HLA-A2 restricted and are currently undergoing clinical testing in the first generation of antigen-specific vaccine studies.
Reactivated embryonic gene products are a second category of malignant melanoma antigens that have been identified. The antigens in this category have been demonstrated to be shared tumor-specific antigens. MAGE1, which was the first gene identified as encoding an antigen recognized by a human melanoma–specific T-cell clone, was found to have a sequence identical to that of the germ-line gene. This gene was found to be expressed in about 50% of melanomas but was not expressed in any adult normal tissue except for the testes. While examples of expression of embryonic gene products in tumors, such as carcinoembryonic antigen and alpha-fetoprotein, have been recognized for quite a while, MAGE1 represents the first such example identified as a tumor-specific antigen specifically recognized by T cells from the patient. Other family members of the MAGE gene have been identified and demonstrate a similar, shared tumor-specific expression pattern.
The third category of identified human malignant melanoma antigens that are the target of T cells is composed of mutated gene products. The best example is the cyclin-dependent kinase 4 (CDK4)-R24C antigen, the result of a mutation in CDK4. The mutation, an arginine-to-cysteine exchange at residue 24, occurs within the antigenic epitope recognized by CD8+ CTLs. In addition, this mutation prevents binding of the CDK4 inhibitor p16INK4a (a tumor suppressor protein), thereby disrupting cell-cycle regulation. T cells specific for other mutated tumor suppressor genes or mutated or rearranged oncogenes have been identified and include the gene products of BCR-abl, mutated ras, and p53. The problem with these particular gene products as targets for vaccination strategies is that in most cases, the site of the mutation or rearrangement tends to vary among tumors, which results in the generation of unique antigenic epitopes derived from commonly mutated proteins. In the case of the CDK4-R24C antigen, the mutation is found in 5% of melanomas tested.
Another important category of commonly expressed potential tumor antigens is represented by viral gene products. It is now known that a number of common human malignancies have viral associations and are indeed characterized by the expression of specific viral gene products. These include the Epstein-Barr virus gene products Epstein-Barr nuclear antigen 1, late membrane protein expressed by nasopharyngeal cancers and some Burkitt's and Hodgkin's lymphomas,HPV E6 and E7 gene products expressed by cervical cancers,and hepatitis B virus gene products associated with some hepatomas.Under certain circumstances, both T-cell and antibody responses against these gene products can be identified.
So far, only one human MHC class II antigen has been identified by techniques that directly use CD4+ T cells. The antigen tyrosinase, which is expressed by more than 50% of human melanomas and encodes for MHC class I-bound epitopes, has been shown to also encode for two HLA-DR4–restricted epitopes. Improved MHC class II antigen isolation methods are needed to facilitate the identification of other human MHC class II antigens. However, there is currently intense debate in the tumor immunology community as to whether the introduction of tumor-associated class II antigens to antigen-specific cancer vaccines will enhance the generation of antitumor immunity.
Significant progress has been made during this decade toward identifying human tumor antigens that are targets of T cells. However, this progress has also raised an important question: Which of the many identified antigens can serve as tumor-rejection antigens in patients? Specifically, the biologic roles of these antigens and their corresponding epitopes in the activation or tolerance induction of T cells and the in vivo rejection of tumors are still not clear.
There is now evidence demonstrating that different antigenic epitopes that derive from the same cellular protein are differentially exposed or hidden from the immune system. Different mechanisms that define the degree of exposure include proteolytic processing, MHC binding on the side of the APC, and T-cell receptor interactions and the T-cell repertoire on the T-cell side. Because many tumor antigens arise endogenously and therefore are a form of self-antigen, those antigens that are most exposed to the immune system may induce the most profound tolerance, which would make them poor tumor-rejection antigens. Therefore, the most likely tumor antigens against which therapeutically relevant immune responses can be induced may be antigens that contain subdominant or cryptic epitopes. Their partially hidden nature may allow T cells specific for them to escape the most stringent forms of tolerance induction, such as anergy or deletion. If this hypothesis is shown to be true, it should even be possible to induce antitumor immunity against the large number of identified nonmutated melanoma antigens, if they are overexpressed and contain hidden epitopes. Thus, knowledge of the most immunologically relevant tumor antigens will have critical implications for the successful development of antigen-specific tumor vaccines.
Vaccines that directly activate T-Cells
One approach to inducing tumor-specific immune responses is to genetically modify tumor cells to express MHC molecules and costimulatory molecules on their cell surface that are critical for T-cell activation. Modification of the vaccinating tumor cell in this way enhances the tumor's ability to directly stimulate T cells. For example, the B7 family of costimulatory molecules, originally described as an activation antigen on B cells, is constitutively expressed by most APCs and is now known to be the ligand for two receptors expressed on T cells. These are CD28 and the CTL-associated antigen-4. CD28 is a critical receptor for generating the costimulatory signals in T-cell activation. Cross-linking of CD28 has been shown to enhance the level of lymphokine production by CD4+ T cells subsequent to antigen recognition.
In animal studies, transfection of B7.1 into some tumors results in rejection of that tumor and generates systemic immunity against wild-type tumor challenges. In other studies, cotransfection of B7.1 with either MHC class II, interleukin (IL)-4, or IL-7 is required to induce potent systemic immunity. The modification of tumor cells to function as professional APCs that directly activate T cells can be accomplished by both in vitro and in vivo gene-transfer strategies. This is also true for cancer vaccine approaches that manipulate professional APCs to enhance tumor-specific T-cell activation.
Cancer Vaccines that attract Professional APCs as facilitators of the antitumor immune response
0A second approach to inducing tumor-specific immune responses is to target the immunizing antigen to professional APCs, which can process and present the antigenic peptide to the T cell in the presence of constitutively expressed costimulatory molecules.Depending on the type of vaccine, targeting of antigens to APCs can be accomplished through a number of different pathways. One central pathway for targeting of vaccinating antigens into bone marrow–derived APCs is the exogenous pathway of antigen uptake. The success of this pathway, operative in most cell-based as well as protein-based vaccines, depends largely upon the adjuvant with which the tumor cell or tumor antigen(s) is formulated. The adjuvant is a critical component of a vaccine. It is the vaccine component that attracts APCs to the sight of vaccination, where they then become activated and take up antigen for processing and presentation.
Although extracts of pyogenic bacteria were used more than 100 years ago, the enhanced understanding of how particular cytokines and other signals mediate differentiation of APCs has led to the design of cancer vaccines that can activate more potent and qualitatively distinct immune responses.
Another pathway for introduction of antigens into appropriate bone marrow–derived APCs is via direct transduction. This can be achieved by certain recombinant bacterial and viral vaccines as well as nucleic acid vaccines, so-called "naked DNA" vaccines. In addition, antigenic peptides can be loaded directly onto empty MHC molecules on the surface of APCs either ex vivo or in vivo, thereby bypassing the processing steps.
Antibody-Directed Vaccines
Apart from direct treatment of cancer with tumor-specific antibodies that are often used to localize various toxins at the tumor site, different active vaccination strategies are being investigated to induce tumor-specific antibody responses. For example, vaccine strategies for the treatment of melanoma were tested using either GM2-expressing melanoma cells or vaccines containing GM2 conjugated to keyhole limpet hemocyanin. These studies demonstrated that vaccines containing purified GM2 ganglioside result in induction of GM2 antibodies, and high titers of GM2 antibodies were correlated with increased survival. One idiotypic vaccine study, which involved the immunization of non-Hodgkin's B-cell lymphoma patients with the unique idiotype of the immunoglobulin expressed on the surface of their tumors, demonstrated a clinical benefit for patients with recurrent B-cell lymphomas. Furthermore, in a preclinical study, an idiotypic immunization with an antibody specific for mutated p53 prevented the development of a p53 mutation–bearing tumor cell line in mice. These encouraging results strongly support the further clinical development of these approaches.
SPECIFIC VACCINE STRATEGIES 15,16,17
Cell-Based Cancer Vaccines
Until the tumor antigens expressed by most tumors are identified, the tumor cell itself will continue to be the best source of immunizing antigens. With the development of improved genetic techniques, the concept of presenting immunologically defined "adjuvants" at the same site as tumor antigens in order to augment antitumor immunity has been tested more directly. In animal models, gene transfer of genes encoding MHC molecules, costimulatory molecules, and cytokines has been studied. Early animal studies evaluated the immune effects of enhanced expression of both autologous and allogeneic MHC class I molecules on the tumor cell surface. Both ex vivo and in vivo gene transfers of these molecules to the tumor have been studied and have met with some success. Similar vaccine strategies are undergoing clinical testing .
Genes that encode cytokines are the most common types of genes that have been introduced into tumor cells. The cytokine is produced at very high concentrations at the vicinity of the tumor, thereby altering the local immunologic environment of the tumor cell so as to either enhance presentation of tumor-specific antigens to APCs or to enhance the activation of tumor-specific lymphocytes. Many cytokine genes have been introduced into tumor cells with varying effects on both tumorigenicity and immunogenicity. Table 2 summarizes the most common cytokines that have been evaluated using this approach and describes the proposed immune mechanisms of tumor rejection generated by these cytokines. Varying results have been reported for certain cytokines, undoubtedly because of the large variations in other critical parameters of immunization. The level of cytokine expression, location of immunization, and challenge site are crucial parameters affecting vaccine efficacy for any form of genetically engineered tumor vaccine. Many of these cytokines have been studied as single cytokines in one or more tumor vaccine models. However, for a human tumor vaccine to be developed, it is critical that these cytokines be compared head to head to determine which cytokine or cytokines are most effective. Also, given that most mouse tumors show significant immunogenicity when simply irradiated, identification of genes that truly enhance the tumor's immunogenicity significantly above that of irradiated wild-type tumor cells is important. Only one study has directly compared multiple cytokines and other genes in murine tumor models.
This study demonstrated, in a number of poorly and moderately immunogenic tumors, that immunization with the tumors transduced with the cytokine granulocyte-macrophage colony-stimulating factor (GM-CSF) produced the greatest degree of systemic immunity, which was enhanced relative to irradiated nontransduced tumor cells.
In vivo depletion of T-cell subsets demonstrated that this immunity was dependent on both CD4+ and CD8+ T cells, despite the fact that the tumors did not express MHC class II antigens. Importantly, tumor cells genetically altered to express GM-CSF were able to cure mice of pre-established small burdens of tumor.
Table 2. Preclinical studies of commonly tested, genetically modified, Cytokine-Secreting Tumor Vaccines.
|
Development and testing of Human Gene–Transduced Tumor Vaccines.
A number of critical immunologic concerns require consideration before human gene–transduced tumor vaccines can be developed. First, human tumors are heterogeneous and therefore probably express many potential tumor antigens. Therefore, one important question to consider is what is the best source of tumor cells that will express these antigens. The best source may be the primary tumor rather than a metastasis, because metastases likely develop from only one or a few clones of cells that arise from the primary tumor. If so, for treatment with an autologous vaccine approach, each patient will require surgical resection of their primary tumor, followed by in vitro expansion and genetic modification to express costimulatory molecules or cytokines. A completed phase I clinical trial evaluating an autologous GM-CSF–secreting tumor vaccine for the treatment of metastatic renal cell carcinoma showed promising results but uncovered a number of technical problems, including the requirement of labor-intensive procedures for production of an individualized vaccine and the difficulty of routinely expanding most primary tumors to the high numbers required for vaccination.These technical limitations might be avoided by using cytokine-expressing bystander cells or cytokine-secreting allogeneic vaccine cells that express shared tumor antigens.
A significant number of clinical studies aimed at augmenting antitumor immune responses against several different cancers have already been completed .The main aim of these studies has been to demonstrate the safety of the genetically modified vaccine. These initial studies have demonstrated that genetically modified tumor vaccines are extremely safe. In fact, the main side effect that has been described is self-limited grade I/II local inflammatory skin changes consisting of erythema and induration at the site of vaccination.
The results of a few of these studies have been optimistic in that they have provided evidence of immune priming as measured by delayed-type hypersensitivity (DTH) reactions to autologous tumor cells. In one study, postvaccination T-cell responses against autologous tumor- and melanoma-specific peptides were observed in vitro. In this same study, an increase in the postvaccination tumor-specific T-cell precursor frequency was also demonstrated. In another study, antimelanoma immunoglobulin G antibody responses were correlated with tumor regression. In a few studies, DTH responses against autologous tumor correlated with regression of distant metastases. In one study, histologic analysis of the vaccine and autologous tumor DTH sites demonstrated immune infiltrates similar to what was seen in animal model testing of the vaccine.These findings suggest a similar mechanism of immune activation against the human tumor as was seen for the murine tumors.
Antigen-Specific Cancer Vaccines
Several antigen-based vaccine strategies have already demonstrated their ability to augment antitumor immune responses in animal models and some are currently undergoing clinical testing. These strategies can be divided into four categories: (1) plasmid DNA–based vaccines that deliver the gene encoding the antigen; (2) recombinant viral and bacterial vaccines; (3) peptide- or protein-based vaccines that deliver the antigen mixed with adjuvants; and (4) antigen-pulsed dendritic cell vaccines.
DNA-Based Vaccines
There has been a renewed enthusiasm to study DNA vaccines because of the surprising finding that plasmid DNA is taken up and expressed by cells in vivo with much greater efficiency than was previously predicted. DNA vaccines are easy to produce in large quantities, thereby providing an advantage over other vaccine strategies. From an immunologic perspective, the unique ability of DNA to either integrate stably into the host's genome or be maintained in an episomal form long-term provides an opportunity for expressing the immunizing antigen for an extended period of time. Successful vaccination using naked DNA against a viral antigen has encouraged an intense effort to explore the possibility of naked DNA as cancer vaccines. Specifically, DNA injection was shown to mediate antibody and cellular immune responses in mice. DNA vaccines are already entering clinical studies for vaccination against infectious diseases and are currently undergoing rigorous testing in animal tumor models. The exact mechanism by which DNA vaccines induce antitumor immunity has not yet been determined. Several studies suggest that immunity is generated by DNA transfection of muscle cells and keratinocytes. More recent studies suggest that bone marrow–derived APCs also play a critical role in inducing antigen-specific immunity.
Intramuscular injections of naked DNA expression plasmids have been shown to generate immune responses . Such DNA vaccines introduce tumor antigen genes into DCs for endogenous processing and presentation to CTLs in draining lymph nodes or into other cells for cross-presentation by DCs, without the need for a viral vector . Thus, problems of competition from viral vector epitopes, reduced efficacy due to prior immunity to the viral vector, and potential dangers associated with a live virus are avoided. Constitutive, tissue, or tumor-specific promoters may be used for selective expression.
Recombinant Viral and Bacterial Vaccines
With the current knowledge of augmenting antitumor immune responses, the ideal antigen delivery vector should (1) directly infect APCs in vivo and (2) facilitate antigen delivery to both MHC class I and II antigen–processing pathways. Several recombinant viral vectors are currently undergoing rigorous testing for their ability to augment antitumor immune responses against model tumor antigens. Of particular interest are vaccinia and other pox viruses and Listeria monocytogenes.
Poxviruses are attractive candidates for the expression of tumor-associated antigens because heterologous proteins are delivered to the cytoplasm and, therefore, are directly targeted to the compartment in which processing of MHC class I antigens is initiated for presentation to CD8+ CTLs. Restifo et al have published several studies demonstrating the generation of antigen-specific immunity resulting in the protection against tumor challenges, using vaccinia and fowlpox constructs. Wu et al more recently demonstrated the enhanced potency of a vaccinia vector carrying the HPV gene E7 fused with the LAMP1 gene, which targets E7 to the MHC class II antigen–processing pathway for presentation to CD4+ T cells. In one study, the cure of a significant tumor burden was demonstrated. A recombinant E6- and E7-expressing vaccinia virus has already been tested in eight patients with late-stage cervical cancer. No significant side effects were described. An HPV-specific CTL response was observed in one of the eight treated patients. However, all eight patients mounted an antivaccinia antibody response. These neutralizing antivirus antibodies represent one of the major barriers to the use of viral cancer vaccines, which can be the result of either previous immunizations (as in the case with vaccinia) or exposure to cross-reactive viruses (as in the case with adenoviral vaccines). It is unlikely that recombinant viral vaccines such as vaccinia will reach broad clinical application until methods have been developed to eliminate neutralizing antibodies or new viral vectors are identified that do not induce significant antibody responses.
L monocytogenes is a novel tumor antigen delivery vector that is particularly interesting because of its two-phase intracellular life cycle, which enables it to deliver antigens to the class I and class II pathways. Recent data have demonstrated the efficacy of using L monocytogenes as a live recombinant vaccine that is sufficiently potent to cause regression of established macrometastases.
Protein and Peptide-Based Vaccines
The aim of protein- and peptide-based vaccine strategies is to administer high doses of peptide that can be loaded onto empty MHC molecules on APCs in vivo. One major advantage of peptide vaccination is that it removes the safety concerns associated with using live recombinant vaccines or DNA vectors. Whereas vaccination with some tumor-associated peptides and proteins has induced systemic antitumor immunity, the administration of other peptides has led to the induction of tolerance rather than activation.
Phase I clinical studies of peptide vaccines have been initiated for the treatment of melanoma. As for whole-cell vaccines, the only toxicities observed have been grade I/II induration and erythema at the vaccine site. One trial of an HLA-A1–restricted MAGE3 peptide mixed with incomplete Freund's adjuvant (IFA) reported three partial remissions in 16 patients with metastatic melanoma at pulmonary and subcutaneous sites. In another study, all three patients with metastatic melanoma demonstrated partial remissions that were associated with DTH reactivity at the vaccine sites after vaccination with three melanoma-associated HLA-A2–restricted antigenic peptides (MART-1/Melan A, tyrosinase, and gp100/Pmel 17) given simultaneously with systemic GM-CSF. A more recent study evaluated an HLA-A2–restricted gp100 peptide analog, which was modified at one of the MHC anchor residues (Thr
Met at position 2) to improve its binding affinity to HLA-A2, in patients with melanoma. Nine patients receiving the unmodified gp100 peptide mixed with IFA were compared with 11 patients treated with the anchor-modified peptide in IFA and 31 patients treated with the anchor-modified peptide in IFA plus systemic IL-2. Immunization with the anchor-modified peptide in IFA induced gp100– and HLA-A2–restricted melanoma CTLs in 10 of 11 patients. Similar in vitro responses were seen in 16% of patients who received the anchor-modified peptide in IFA together with systemic IL-2.

Although clinical responses were not observed in patients who received peptide plus IFA without concomitant IL-2, there was a 42% response rate (1 complete remission, 12 partial remissions) among patients who received the same vaccine along with systemic IL-2. It is not clear from this initial study whether the peptide and IFA vaccination contributed to the clinical responses because an IL-2–only treatment group was not included. Furthermore, prospective randomized clinical studies comparing vaccinated patients with unvaccinated patients are needed to determine whether the responses seen in phase I testing are due to the vaccine or the natural biology of malignant melanoma.
A novel peptide vaccine approach uses heat shock proteins (HSPs). HSPs, which are found in most cells and can bind a wide variety of peptides, are being tested as natural adjuvants for their ability to elicit antitumor immune responses. Immunization with tumor-derived HSP96, an endoplasmic reticulum resident, and HSP70, a cytosolic HSP, generates tumor-specific antitumor immunity in mice. This tumor-specific immunity is mediated by specific peptides, which are found bound to HSPs. It is postulated that HSPs induce a specific CTL response by introducing antigens into the MHC class I and II pathways as well as by binding to macrophages, thereby inducing the production of proinflammatory cytokines.
Dendritic Cell Vaccines
Dendritic cells, which were originally isolated and characterized by Steinman and Cohn in 1973, are currently the most potent APCs that have been identified. Activated bone marrow–derived dendritic cells express high levels of MHC class I and II molecules on their cell surface as well as intercellular adhesion molecule and B7, two important adhesion and costimulatory molecules required for T-cell activation.
Recently, several dendritic cell growth factors have been identified that have facilitated their in vitro expansion and activation, allowing for the in vitro priming of dendritic cells with specific antigens. On the basis of these findings, several approaches
using dendritic cells have been tested in animal models, including dendritic cells loaded in vitro with peptides or protein, dendritic cells fused with whole tumor cells, and ex vivo transduced dendritic cells. Most of these studies have demonstrated rejection of significant tumor burdens.
Clinical trials of DC vaccines have depended on development of techniques for obtaining large numbers of clinical grade human DCs Currently, two general approaches are used: (a) purification of immature DC precursors from peripheral blood ; and (b) ex vivo differentiation of DCs from CD34+ hematopoietic progenitor cells or peripheral blood monocytes , commonly by culture of monocytes with GM-CSF and IL-4 . Immature DCs can be matured with CD40 ligand, LPS, or TNF-α . In mice, CD40 ligand–mediated maturation was the most effective approach for DC vaccine preparation .
The first clinical trial testing peptide– and tumor lysate–pulsed dendritic cells in 16 patients with metastatic melanoma has recently been completed. Regression of metastases in various organs (skin, soft tissue, lung, and pancreas) was observed in five patients. No physical signs of autoimmunity were detected and the vaccinations were well tolerated. Another clinical trial used dendritic cells pulsed with tumor-specific idiotype protein for patients with follicular B-cell lymphoma. All four patients in this study developed a measurable immune response, and one patient experienced tumor regression.
Ganglioside based vaccines.
The gangliosides comprise a family of more than thirty complex glycosphingolipids, which have one or many Nacetylneuraminic acid residues (also known as sialic acid). They are abundant in the neuron's plasmatic membrane, although they are present in most cellular types in much smaller quantities. The ganglioside antigenic presentation, as all other synthetic or bacterial glycolipids, is not mediated by classic MHC molecules, but by a family of presenting molecules called CD1 . Although the function of most gangliosides is not completely understood, it is known that some of them are involved in interaction processes between the cell and its microenvironment, potentially be involved in angiogenesis and metastatic growth regulation, and they may also play a role on tumor-induced immunosuppression.
MEB4 melanoma cells pharmacologically depleted of gangliosides (mainly GM3) by treatment with a specific glucosylceramide synthase inhibitor, showed a marked reduction in their ability to form tumors as well as a great reduction in the metastatic potential, proving that gangliosides are important factors in both tumor and metastasis growth . A systematic study on ganglioside expression in a group of melanomas and normal tissue identified GD3 as another ganglioside prominent in neoplastic tissue. GD3, as GM3, however, was also detectable in normal tissue. GM2 and GD2, on the other hand, are present in most melanomas, but their expression in normal tissue is restricted to the brain . Another factor to fuel interest in GM3 as immunotherapeutic target has been the recent discovery that chemotherapyresistant cancer cells have higher levels of this ganglioside.
Heat Shock Proteins (HSPs)
HSPs were purified from tumor cells and shown to provide protective immunity in animals in 1984 . Autologous HSP based immunotherapy studies in humans started in 1995 in Berlin and 1997 in New York . As of 2003 _500 patients with eight different types of cancer have been treated . The gap between the first observation in laboratory animals and human clinical trials is a testament to the intensive efforts.
This body of work is a noteworthy example of translational biology where remarkable efforts to understand a powerful immunological phenomenon have led to advanced clinical testing in humans.
HSPs as Chaperones
Because HSPs were known to be chaperones, aiding in the transport of peptides throughout the cell, it was proposed that HSPs isolated from tumor cells contain low-molecular-weight antigenic peptides and that these HSP–peptide complexes conferred protective immunity to cancer. Several lines of evidence corroborated this hypothesis, the most important of which has been the isolation and identification of antigenic peptides stripped from purified HSP preparations of tumor cells. The peptides were shown to be of a large variety and included cytotoxic T cell epitopes of the cancer It was further demonstrated that HSP preparations treated to unbind peptides were not immunogenic. Thus, in cancer the specific immunogenicity of the HSP preparations can be attributed to the unique repertoire of antigenic peptides that exists in different cancers. The unique peptide repertoire is a product of mutations in cancers, and as mutations arise randomly, this repertoire is highly likely unique to each cancer. The HSP–peptide complexes thus confer specific immunity only to the cancer from which they are isolated.
HSP Immune Biology
HSPs have been demonstrated to activate CD8_ and CD4_ lymphocytes; induce innate immune response including natural killer cell activation and cytokine secretion; and induce maturation of dendritic cells . It is perhaps apt therefore to call HSPs the ‘‘Swiss army knife’’ of the immune system . These activities are likely important in immune rejection of cancer. The early discovery that animals could be specifically vaccinated against autologous cancers led several investigators to search for the molecules within tumor cells that might be responsible for conferring immunity. Tumor cell lysates were fractionated biochemically, and individual fractions were tested for activity in tumor protection experiments in vivo. The protective fractions were observed to contain HSPs .
Consistent with the early demonstration that immunity generated with whole tumor cells is tumor specific, it was observed that HSPs elicited immunity only to the tumor from which they were purified. Tumor rejection activity has been seen with several different HSPs in multiple cancer models . When mice with bulky metastatic lesions are treated with HSPs, what is typically observed is a slowing of the rate of tumor growth or stabilization of disease.
Generally, only a small proportion of the animals achieve complete tumor regression. In contrast, HSP treatment of mice with resected primary tumors or minimal residual disease confers long-lasting protection from recurrence of tumors and the vast majority of animals live their normal life span . The finding that HSPs elicit specific cancer immunity is ironic because HSPs are among the most highly conserved proteins in invertebrate and vertebrate biology. How were these molecules, which are ubiquitous and so similar in structure and sequence among species, able to elicit specific cancer immunity? Adding to the mystery was the finding that no differences in gene sequences were observed between HSPs isolated from normal tissues and tumor tissues. Immunity elicited by HSP vaccination was shown to require professional antigen-presenting cells (APCs) and CD8_ T cells because depletion of either cell type abrogates protection. The efficiency with which HSPs elicit immune response suggested a receptor-mediated mechanism of HSP uptake by APCs, and such receptor was recently identified as CD91 . Despite their exogenous route of administration, HSP–peptide complexes prime CD8_ T cell responses, suggesting that HSP-chaperoned peptides gain access to the MHC classI antigen processing pathway in the immunized host’s dendritic cells and macrophages.
In studying the interaction of purified HSP preparations with various immune cells in both murine and human systems, it became clear that HSPs elicit a variety of innate immune responses that are independent of chaperoned peptides. These responses include cytokine and chemokine release by antigen presenting cells and T cells, maturation of dendritic cells, and induction of migration of dendritic cells to draining lymph nodes. Several studies suggest that this innate immune response elicited by HSPs contributes to cancer immunity; however, the strongest anti-tumor effects are observed upon concurrent priming of tumor-specific T cells by HSPchaperoned tumor-specific peptides.
Antigen/adjuvant vaccines
Antigen vaccines were some of the first cancer vaccines investigated. Antigen vaccines commonly use specific protein fragments or peptides to stimulate the immune system to fight tumor cells. One or more cancer cell antigens are combined with a substance that causes an immune response, known as an adjuvant. A cancer patient is vaccinated with this mixture. It is expected that the immune system, in responding to the antigen-carrying adjuvant, will also respond to tumor cells that express that antigen.
Idiotype vaccines
Since antibodies are molecules containing protein and carbohydrate, they can themselves act as antigens and induce an antibody response. Antibodies produced by certain cancer cells (i.e., B-cell lymphomas and myelomas), called idiotype antibodies, are unique to each patient and can be used to trigger an immune response in a manner similar to antigen vaccines.
CLINICAL TRIALS 20,21
AG-858 personalized cancer vaccine
AG-858 (HSPPC-70) is an investigational personalized vaccine designed to treat cancer while minimizing side effects that are often associated with other treatment options. AG-858 is currently being investigated as a cancer treatment and is only administered to patients in research studies.
AG-858 is a vaccine made from individual patients’ cancerous cells. Patients undergo a blood filtering process called leukapheresis, during which white blood cells are collected. The white blood cells are sent to Antigenics so that the personalized vaccines can be made.Vaccines provoke the body’s natural disease-fighting response. A vaccine that is made from the patient’s own cancerous cells is designed to contain the particular cancer’s ‘fingerprint.’ Injection of the AG-858 vaccine may cause the body to attack any cells bearing this cancer fingerprint.
In the trial of AG-858 in chronic myelogenous leukemia, white blood cells from the patient are sent to Antigenics, where the personalized vaccines are made. Using a standardized quality-controlled process, heat shock proteins and their associated peptides are isolated from the cells and made into an injectable vaccine. To minimize disruption to the patient’s everyday life, AG-858 treatment is administered on an outpatient basis over the course of several weeks.
AG-858 is currently being evaluated in combination treatment for chronic myelogenous leukemia (CML). Based on findings from an ongoing pilot study being conducted at the University of Connecticut of an earlier HSP70-based vaccine in combination with the FDA-approved therapy Gleevec® (imatinib mesylate, Novartis), Antigenics is conducting an exploratory Phase 2 study of AG-858 in combination with Gleevec for the treatment of chronic phase CML.
Improved Quality of Life for Kidney Cancer by Oncophage® (HSPPC-96) Cancer Vaccine.
This observational study measured quality of life in patients with newly diagnosed, stage IV renal cell carcinoma (kidney cancer) who were participating in a clinical trial of the Oncophage cancer vaccine. In the trial, patients received four weekly injections of personalized Oncophage vaccine once they had recovered from surgery. Quality of life measurements were taken at the start of Oncophage treatment, three weeks later on the final day of treatment, and at follow-up one month later (approximately 13 weeks after surgery). The patients’ self-reported assessments of various aspects of quality of life, such as physical functioning, bodily pain, fatigue, energy, social functioning and mental health, were compared with assessments self-reported by the general population and by patient groups with chronic diseases.
The study showed that quality of life remained stable or improved during treatment with Oncophage. By the one-month follow-up assessment, the patients’ physical functioning assessments had improved significantly, although they remained significantly lower than those reported by the general population. The study also found that the quality of life of the enrolled patients was comparable to that of the general population on psychosocial and emotional dimensions such as vitality, social function, mental health, and role limitations due to emotional problems. Overall, the quality of life reported by patients in the study was similar to that reported by patients with type 2 diabetes, and significantly better on four dimensions (physical function, general health, vitality, role limitations due to emotional problems) than that reported by patients with congestive heart failure.
Approximately 700 cancer patients in more than a dozen clinical trials around the world have received Oncophage in clinical trials. Many of these patients had advanced disease, including kidney cancer, melanoma and colon cancer, and had not responded to traditional cancer treatments.
POSSIBLE BENEFITS OF CANCER VACCINES
In addition to providing a new treatment option for patients who have failed other therapies, clinical data suggest that cancer vaccines may offer therapeutic advantages over existing therapies.
1. Favorable Side Effect Profile: Unlike many traditional cancer treatments such as chemotherapy and radiation therapy, cancer vaccines have generally been associated with very few side effects. This favorable side effect profile may potentially enable patients to maintain a higher quality of life during the course of treatment.
2. Combination Therapy: Numerous clinical trials are being conducted evaluating the use of cancer vaccines in combination with other traditional therapies such as chemotherapy, radiation therapy, and stem cell transplantation. Combination therapies offer the potential of improving/enhancing the efficacy of these traditional treatments.
CHALLENGES FACING 22
The problem with cross-reactivity.
Cancer vaccines need to be directed at antigens that are expressed by the tumor but not by the normal cells. If the antigen that is used is also expressed in normal cells, the immune response generated by the vaccine can attack normal tissue as well. This cross-reactivity can increase the toxicity of the treatment, interfering with one of the biggest potential advantages of cancer vaccines: the ability to specifically target the cancer cells without damaging the normal cells.
Cancer cells inhibit co-stimulation of the immune response.
Co-stimulation of the immune response must occur for there to be a vigorous immune response. Tumor cells secrete chemical factors that interfere with co-stimulation of the immune response. Even if an APC is able to present antigens to T-cells, the immune response is often limited without co-stimulatory signals. Cancer vaccines that present the TAA to the immune system without increasing co-stimulation run into the same problem. The immune response is often too weak for any significant effect on the tumor to occur. In order to solve this problem, cancer vaccines are often designed to provide co-stimulation in addition to the TAA in order to increase the immune response.
The larger the tumor, the more difficult it is to destroy all of the cancer cells.
When individual cancer cells are found, the immune system can easily clear them from the body. Unfortunately, by the time most cancer vaccines are given, the tumors are large and much more difficult to treat. In addition to the sheer number of cells that need to be destroyed, as tumors grow, there are areas within the tumor that become difficult for the immune system to reach. These areas become protected from the effect of the cancer vaccine.
The immune system slows down as we age.
The thymus is an organ in the chest that functions to produce T-cells (the T stands for thymus). It functions in childhood and slows down with time, eventually becoming inactive in adults. This is one of the reasons that vaccines are often given in infancy and childhood. The immune response of adults is dependent on the population of T-cells that was produced during childhood. Over time, this response becomes less vigorous. Cancer vaccines face the challenge of inducing immune responses in a population of patients whose immune systems are naturally slowing down.
Cancer cells themselves are immunosuppressive.
Cancer cells can suppress the immune system in a number of ways. They can block co-stimulation, preventing a vigorous immune response. Cancer cells can inhibit the maturation or function of APCs, blocking the presentation of TAAs to lymphocytes. In addition, cancer cells can directly block the activation of lymphocytes, preventing a response after the antigens have been presented.
DO CANCER VACCINES WORK?
There have been a number of clinical studies that have tested cancer vaccines. Thus far, no trials have found that cancer vaccines improve overall survival. However, a number of important findings have come out of these studies:
1. Cancer vaccines are well-tolerated
A number of Phase I and II trials have shown that cancer vaccines are generally well-tolerated, particularly with the viral and peptide vaccines. Phase I trials have reported very few significant toxicities with these vaccines. The most common toxicity in all of these trials has been local irritation at the site of the injection. In fact, it appears that patients that have a stronger local reaction to the injection may have a better response of the tumor to the treatment. This may be because a local reaction serves as a marker for people who have a good immune response to the vaccine. Patients with strong local reactions will likely have a strong reaction to the tumor as well.
2. Cancer vaccines induce an immune response
In both Phase I and Phase II trials, levels of immune activity have been measured in patients receiving cancer vaccines. Routinely, objective measures have shown an increase in the immune activity in a number of patients receiving cancer vaccines. In addition, patients who have a good immune response by objective measures have a better response of their tumors to the vaccine.
3. Several cancer vaccines show a good tumor response compared to historical results
Recently, the results of a non-randomized trial utilizing a melanoma vaccine were reported. Patients receiving the vaccine had a 5-year overall survival of 44%. Given the advanced disease in these patients, these results were quite good, and noticeably higher than historical surgical series of patients with similarly advanced disease showing 5-year overall survival rates of 20-25%. Another promising trial was recently reported which used a prostate cancer vaccine in patients with progressive disease (judged by rising PSA levels) after local therapy for prostate cancer. After a year and a half, 45% of patients did not have further increases in their PSA.
4. Results of Phase III, randomized trials have been disappointing
Despite the promising results in earlier trials, several large randomized trials have failed to show an overall survival advantage with the use of vaccines. A large trial by the Southwest Oncology Group for patients with melanoma who did not have spread of their disease to the lymph nodes showed no improvement in overall survival for patients who received the vaccine following surgery compared to patients who underwent surgery alone. Another trial for patients with renal cell carcinoma showed a small decrease in the percentage of patients who had progression of their cancer with the addition of a cancer vaccine. 14 Again, however, there was no improvement in overall survival with the use of the vaccine.
It is becoming clear that the immune response may be hindered by the hurdles created by tumors to evade the immune system. As noted, VEGF and other tumor-derived factors can inhibit DC maturation, and maturation of DCs ex vivo can circumvent this roadblock. We also discussed under “Peptide vaccines” (above) the use of cytokines, chemokines, and costimulatory molecules to activate and direct the immune response toward the appropriate type, as well as epitope enhancement to improve binding of tumor antigen epitopes to MHC molecules or TCRs.
Another area generating much recent interest is the possibility of overcoming mechanisms that downregulate or attenuate the immune response . Such mechanisms may have evolved to reduce inflammation and immunopathology or to prevent autoimmunity. Tumors have co-opted these mechanisms to evade immunosurveillance. T cells themselves express inhibitory receptors, the best studied being CTLA-4 . This binds costimulatory molecules CD80 and CD86, but, instead of activating the T cell, dampens its response. Blockade of CTLA-4 has been shown to improve tumor immunosurveillance and amplify effects of cancer vaccines in animals . Recently, monoclonal anti–CTLA-4 antibodies have been studied in clinical trials alone or in conjunction with cancer vaccines . A substantial number of objective responses were found in a melanoma trial, but not without a number of autoimmune side effects, all reversed when therapy was stopped
Another regulatory mechanism is the CD25+CD4+ regulatory T cell . Such cells are induced by antigens, especially in the presence of high IL-2 levels, but their effector activity is not antigen-specific . Blockade or elimination of these cells has been shown to enhance tumor immunosurveillance and efficacy of antitumor vaccines . Furthermore, concurrent blockade of
CD25+CD4+ regulatory T cells and of CTLA-4 was synergistic in augmenting efficacy of a cancer vaccine .
Another novel immunoregulatory cell is the CD4+ NKT cell. These are T lymphocytes that share certain markers with NK cells but also express normal CD3 and αβ TCRs. However, the TCRs used are limited to a few types that predominantly recognize glycolipids presented by a nonclassical class I MHC molecule, CD1d . These cells play a role in regulating immune responses causing autoimmune diseases, such as diabetes . Recently, we found that CD4+ NKT cells also can inhibit tumor immunosurveillance in a mouse fibrosarcoma model and an orthotopic breast cancer model , and have now extended these findings to a non-regressor colon cancer model (Park et al., unpublished results) . Elimination of NKT cells, or blockade of their effector mechanisms, such as IL-13 or downstream TGF-β, prevented tumor recurrence and improved CTL responses and antiviral efficacy of a peptide AIDS vaccine in mice . Whereas regulatory NKT cells express predominantly Th2 cytokines, other NKT cells making IFN-γ can actually contribute to tumor immunosurveillance . Factors influencing these opposing roles of NKT cells are under study. Thus, we propose that blockade of NKT cell negative regulatory cytokines can be used as a strategy to increase the efficacy of anticancer vaccines .
A complementary approach is to selectively induce high avidity CTLs , which were first shown to be more effective at clearing virus infections and then also to be more effective at killing tumor cells and eradicating tumors . We recently found that increasing levels of costimulation, using a triad of costimulatory molecules — CD80, ICAM-1 and LFA-3 — in conjunction with a vaccine, can selectively induce CTLs skewed toward higher avidity and more effective at killing tumor cells . Moreover, IL-15 expressed by a vaccine has also been found to selectively induce longer-lived CTLs that may be more effective and also a higher average avidity of CTLs (Oh et al., unpublished results). Indeed, we suggest that the recently discussed role of CD4+ T cell help and signal strength in inducing long-lived memory CD8+ CTLs may be mediated in part by helper cell stimulation of DCs to produce IL-15 when they present antigen to CTLs, as we have mimicked with IL-15 in the vaccine . These strategies may be useful in optimizing anticancer vaccines
FUTURE HOLD FOR CANCER VACCINES
Cancer vaccines remain an important and growing area of cancer research. A number of phase III trials are currently underway to further evaluate the effectiveness of these vaccines in improving patients' outcomes. A number of key questions and areas of research remain:
- Can we improve the specificity of cancer vaccines through the identification of new tumor-associated antigens or possibly through the engineering of known antigens?
- Can we improve the strength of the tumor response, possibly through improving co-stimulatory signals?
- Will new, alternative methods of delivering antigens to the immune system improve the immune response?
- Will cancer vaccines be more effective against earlier stage cancers where the amount of tumor is less?
- Can combining cancer vaccines with other treatments such as chemotherapy and radiation improve patients' outcomes?
- Will cancer vaccines ever be shown to improve the overall survival of cancer patients?
We are still quite a ways away from incorporating cancer vaccines into the routine care of cancer patients. Nevertheless, the possibility of using the body's own immune system to destroy cancer cells remains an appealing possibility and results of early trials are promising. Research into new ways of treating cancer, such as cancer vaccines, remains critical in our ongoing fight against cancer.
OUTLOOK
Recent advances have made possible the design of recombinant vaccines that more effectively augment antitumor immune responses in preclinical models. Many of these strategies are already undergoing initial clinical testing. The availability of tumor antigens defined at the genetic level will provide new opportunities for recombinant vaccine design. However, several issues require consideration during the early phases of clinical testing. First, although many promising vaccine strategies have been demonstrated in animal models, it seems inefficient and a waste of limited resources to rigorously test each one separately in patients. Most of these vaccines are expensive to produce and require labor-intense development for scale-up to treat large numbers of patients. A head-to-head comparison of vaccine strategies demonstrated to be most potent in animal models would help to limit the focus. Similarly, as has been demonstrated for melanoma, many tumor antigens can serve as T-cell targets expressed by tumors. Yet, it is a time-consuming process to test each antigen for its potential as an immunogen in patients with cancer. Preclinical models are needed to determine which antigens require expedited testing in clinical trials. Second, the optimal dose, route, and schedule of administration for each vaccine strategy is usually not known because it is difficult to address these questions in animal models in which the doubling time of a growing tumor mass is 48 to 72 hours. Therefore, these questions must be addressed in early clinical trials.
Several hurdles must be overcome. First, it has been demonstrated that cancer cells can undergo genetic alterations that result in their ability to evade immunologic recognition and eradication, either because of loss of antigen expression or loss of the ability to present the antigen to T cells. One possible solution to this problem is to design polyvalent vaccines that target immunity against several antigens. A second possibility is to treat patients with early stages of disease before the tumor population has a chance to undergo significant mutations. A third possibility is to combine vaccines with other cancer treatment modalities. Second, the identification of tissue-specific antigens as a major category of tumor antigens that serve as T-cell targets has generated significant concern that vaccination might result in significant autoimmune toxicities. However, as we learn more about the mechanisms of immune activation and regulation, it should be possible to incorporate strategies for immune regulation into the overall cancer vaccine treatment plan.
Although caution is needed, there are many promising vaccine approaches that require testing. Ultimately, the development of multiple strategies that can be applied in synergy will most likely be responsible for the major advances against cancer. It is too early to see what place immunotherapy in general will have in the fight against cancer. However, it is clear that it is now feasible to design vaccines that are based on rational immunologic principles.
CONCLUSION
Immune therapy of cancer may take many forms, specific or non-specific, adoptive or active, and may target antibody, T cell, and innate immune mechanisms. Each of these approaches has proven or potential value, and the complexity of the host: tumor relationship is such that a narrow focus on a single immunotherapy strategy is likely to fail. Adoptive T cell immunotherapy studies have provided strong proof of principle that antigen-specific CD8+ T cell responses to cancer can mediate dramatic cancer regressions. However, adoptive therapy is cumbersome and expensive, and difficult in the current regulatory environment. Vaccines, on the other hand, are more readily adaptable for therapy outside of highly specialized centers. In particular, peptide vaccines are easily produced, standardized, and administered. The current appeal of adoptive therapy is that antigen-specific T cells can be expanded and activated at high numbers ex vivo, more readily than they can be expanded in vivo in cancer patients. However, we argue that the lesion in current approaches to cancer vaccine therapy is our poor understanding of the mechanisms that limit expansion, activation, and effector function of tumor-antigen specific T cells. Bypassing this process by use of adoptive therapy is a reasonable short-term effort, but ultimately to advance the field of tumor immunology and immunotherapy it will be critical to elucidate the immunobiology of the host-tumor relationship. Appropriate design of cancer vaccines using multiple antigens should be combined with careful monitoring of T cell expansion and T cell function. Optimally, immune monitoring should be performed in multiple compartments (peripheral blood, tumor tissue, lymph nodes). The next wave of investigation in cancer immunotherapy has begun, and will include combination therapies designed to activate innate and adaptive immunity simultaneously and to down-modulate tumor-associated immune regulation. Vaccines with defined antigens are ideal for investigations of this type.cb
No comments:
Post a Comment
leave your opinion