
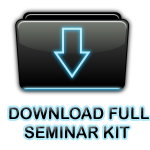
INTRODUCTION
Tsunami is a natural disaster. It cannot be avoided.But we can reduce the damages caused by it. For that we are in need of tsunami warning system. The system used here is Deep-ocean Assessment and Reporting of Tsunami(DART).We are also going to see how the global system for mobile communications(GSM)network reduces the time taken to warn people.
TSUNAMI FEATURES
In the open ocean, tsunamis may have wavelengths up to several hundred miles and travel at speeds up to 950 kilometers per hour-as fast as a passenger jet. The speed of tsunami is controlled by water depth-as the wave approaches land it reaches shallow water and slows down .Compared to the front of the wave, the rear moves slightly faster and catches up. The result is that the wave quickly ‘bunches up’ and becomes much higher. the highest tsunami occur if they encounter a long and gradual swallowing of the water, because this allows enough time for the wave to interact with its surroundings and cause extensive damage to low lying areas.
TSUNAMI WARNING SYSTEM
Tsunamis strike without warning .The resulting damage can be maintained and lives can saved if the people living near the coastal areas are already prepared to survive the strike. This requires a warning system .The type discussed here is Deep ocean Assessment and Reporting of Tsunamis {DART}.Each DART station consists of a seafloor Bottom Pressure Recording {BPR} package that detects pressure changes caused by tsunamis and a surface Buoy which receives transmitted information from BPR via an acoustic link. The Buoy and BPR together are called a “TSUNAMETER” and each one costs about USD 250,000.
The BPR monitors water pressure with a resolution of approximately 1mm of sea water with 15-second averaged samples. Data are transmitted from the BPR via an acoustic modem and data are transmitted from the buoy via the GOES data collection system. Under normal conditions the BPR sends data hourly that is comprised of four 15-minute values which are single 15-second averages. The BPR can make up to 3 tries to get acknowledgement from the surface buoy that the data were received. The data are reformatted and send via the GOES self-timed channel and displayed at the NDBCDART page to show open tides. The hourly observation indicates the health and condition of the entire system. If data are not received from the BPR, the surface buoy uses the GPS derived buoy position for self timed message. An algorithm residing in the BPR generates predicted water height values and compares all new samples with predicted values.
TSUNAMI DETECTION ALGORITHM
Each Deep ocean Assessment and Reporting of Tsunamis (DART) gauge is designed to detect and report tsunamis on its own, without instruction from land. The tsunami detection algorithm in the gauge’s software works by first estimating the amplitudes of the pressure fluctuations within the tsunami frequency band and then testing these amplitudes against a threshold value. The amplitudes are computed by subtracting predicted pressures from the observations, in which the predictions closely match the tides and lower frequency fluctuations. The predictions are updated every 15 seconds, which is the sampling interval of the DART gauges.
Background oceanic noise determines the minimum detection threshold. Based on passed observations, a reasonable threshold for the north pacific is 3cm. if the amplitudes exceed the threshold; the gauge goes into a rapid reporting mode to provide detailed information about the tsunami. It remains in this mode for at least 4 hours.
FORM OF THE TSUNAMI DETECTION ALGORITHM
The tides and lower frequency signals are predicted within a few millimeters using a cubic polynomial that is fit to Bottom Pressure Observations over the past3 hours.
Hp (t’) =w (i)H*(t-idt)
Where the asterisk denotes 10-minute averages and dt =1hour. The prediction time t’ is said to 5.25 minutes, which is half the 10 minute averaging interval plus the 15 seconds sampling interval for the gauges. The coefficients w (i) come from Newton formula.(II) for forward extrapolation. Using these temporal parameters the w-coefficients are
W (0) =1.16818457031250
W (1) =-0.28197558593750
W (2) =0.14689746093750
W (3) =-0.03310644531250
A tsunami is detected if the difference between the observed pressure and the prediction Hp exceeds the prescribed threshold in magnitude (30mm in north pacific). The gauges could use the most recent pressure observation to test against prediction. However, the next earlier is used so that the gauges can screen the pressure values for instrumental spikes that might falsely trip the algorithm. The threshold for these spikes is set at 100mm.
THEROTICAL PRESSURE SERIES
The graph shows the application of the algorithm to a theoretical pressure series. The series consist of an M2 tide with amplitude of 1mm and a short pulse that has amplitude of 5cm and duration of 15 minutes. The pulse affects the difference both directly and through its indirect effect on the prediction. Following the first and largest differences, pulses continue to occur each hour with diminishing amplitude until the pulse no longer influences the predictions.
The difference exceeds the threshold at the beginning of the theoretical series. This is due to the mismatch between the time series and the constant values placed initially in the H*array. This phenomenon will also occur during field deployments of DART gauges as they fall through the water column towards the bottom.
However, the difference will stabilize at sub-threshold values 4-5 hours after the gauges reach the bottom. Then, the H* array contains only on-bottom pressure values, and the gauges are in thermal equilibrium with the bottom waters. As shown in fig 1, a software flag is set to -1 each time the difference exceeds the threshold. In turn, this exceedance flag controls a reporting flag that puts the DART gauge into
its rapid reporting mode. The reporting flag is set to -1 as soon as the threshold is exceeded and remains equal to -1 until 4 hours has passed since the last time the threshold was exceeded. The gauge then returns to its monitoring mode.
THE FREQUENCY RESPONSE
From the graph, the pressure difference has a response near unity over the tsunami band, which spans the periods from 2-90 minutes. At lower frequencies, the attenuation
Increases rapidly with decreasing frequency. Hence, tsunami and higher frequency signals dominate the pressure difference.
OBSERVED TSUNAMI
From the above graph, small amplitude tsunamis observed by internally recording gauges in the north pacific. Relative to the high-passed data, the background noise in the difference series is either equal in amplitude or slightly enhanced. Neither tsunami high enough in amplitude to set a DART gauge into rapid reporting mode.
COMMUNICATING THE DANGER
Time taken to warn people by conventional method is too high. So we have devised a method using GSM technique. This GSM based alert system monitors the sea waves using the Tsunameter and broadcasts an alert message to towns and villages through the cell phone network existing throughout the state. Here the mobile phones are used both as transmitters and receiver. This is only an alert network and does not find the location of the epicenter, only the discreet magnitude levels will be detected and any magnitude above the preset threshold level will be transferred to the mobile receiver handset via Short Message Service (SMS). The handset, in turn, will transfer it to Base Transceiver Stations (BTS) if it is within 10-17 km of BTS. Otherwise it may require a repeater for transferring data to cell phone network.
The handset should have auto- dialing facility and be preloaded with a fixed dialing number and fixed message. Whenever the handset gets a signal from the interface unit , it goes into the auto dialing mode and transfers data to the network and waits for the next pulse with the same number and the message.
DECISION SYSTEM
When SMS is sent from the mobile handset, it first goes to the SMS of the mobile network, then to the destination receiver handset. If the same has to be sent to the different locations (receivers), the transmitter handset has to dial different number for different destination.
But on this proposed system, on a single transmission the message should go to different predefined locations. This is possible if the SMS server of the mobile network is programmed for such a facility. In fact, this type of facility already exists in the GSM network in the form of ‘cell broadcast’. But it is not advisable to disturb the main server for this. Another application server may be included for this purpose. The SMS server sends 40 messages per second to all destinations through the GSM network. Therefore in this mode it takes a maximum of only one second for sending the alert signals to the predefined locations.
Since the pressure level of normal sea waves and tsunami waves are different, this system sends an alert message only if the pressure level exceeds a threshold. The magnitude threshold for sending the alert signals can be decided by an experts committee.
CONCLUSION
The Tsunami warning center senses tsunami, transits these discrete magnitude values to central place via GSM cell phone network, and uses computer-based decision making to deliver alert signals to the identified receivers placed at different towns and cities for both public and government consumption.
The system is simple and could be configured with available resources .here only concepts are discussed .detailed simulation, feasibility study and experimentation are required to optimize the system and reduce the possibilities of false alarm.
No comments:
Post a Comment
leave your opinion