
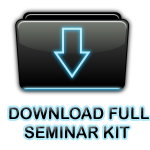
Since this definition is good for most porous materials, the term aerogels became reserved for the porous gels obtained by removing solvent from highly swollen gels at the conditions that no or minimal collapse occurs, which causes the liquid in the gel to become supercritical (in a state between a liquid and a gas) and lose its surface tension. The result is an open porous material with a backbone morphology that can be modeled in terms of three dimensionally interconnected strings of nanoscopic pearls. The length scale of both the “pearls” as well as the interconnected voids can be independently tailored over a wide range, i.e. from a few nanometers to several microns.One of the striking advantages of aerogels compared to other porous materials is that both porosity and inner surface area can be tuned independently. Porosities of up to 99.9 % are achievable; when microporosity is present, the specific surface area can exceed 1500 m2/g. Because of their unique properties, i.e., large surface area, very small pores and very low bulk density, aerogels are potentially important candidates for a wide range of applications.
HISTORY
Steven. S. Kistler of the College of the Pacific in Stockton, California set out to prove that a "gel" contained a continuous solid network of the same size and shape as the wet gel. It is believed that Kistler's interest was stimulated by a friendly wager with fellow worker Charles Learned. They competed to see if one of them could replace the liquid inside a jelly jar with gas without causing any shrinkage. Kistler won the bet, and published his findings in a 1931 edition of the journal Nature.
As is often the case, the obvious route included many obstacles. If a wet gel were simply allowed to dry on its own, the gel would shrink, often to a fraction of its original size. This shrinkage was frequently accompanied by severe cracking of the gel. Kistler surmised, correctly, that the solid component of the gel was microporous, and that the liquid-vapor interface of the evaporating liquid exerted strong surface tension forces that collapsed the pore structure. Kistler then discovered the key aspect of aerogel production:
Aerogels had been largely forgotten when, in the late 1970s, the French government approached Stanislaus Teichner at Universite Claud Bernard, Lyon seeking a method for storing oxygen and rocket fuels in porous materials. There is a legend passed on between researchers in the aerogel community concerning what happened next. Teichner assigned one of his graduate students the task of preparing and studying aerogels for this application. However, using Kistler's method, which included two time-consuming and laborious solvent exchange steps, their first aerogel took weeks to prepare. Teichner then informed his student that a large number of aerogel samples would be needed for him to complete his dissertation. Realising that this would take many, many years to accomplish, the student left Teichner's lab with a nervous breakdown. Upon returning after a brief rest, he was strongly motivated to find a better synthetic process. This directly lead to one of the major advances in aerogel science, namely the application of sol-gel chemistry to silica aerogel preparation. This process replaced the sodium silicate used by Kistler with an alkoxysilane, (tetramethyorthosilicate, TMOS). Hydrolyzing TMOS in a solution of methanol produced a gel in one step (called an "alcogel"). This eliminated two of the drawbacks in Kistler's procedure, namely, the water-to-alcohol exchange step and the presence of inorganic salts in the gel. Drying these alcogels under supercritical alcohol conditions produced high-quality silica aerogels.
PHYSICAL PROPERTIES OF SILICA AEROGEL
Property | Value | Comments |
Apparent density | 0.003-0.5 g/cm3 | Most common density is 0.1g/cm3 (air = 0.001g/cm3) |
Inner surface area | 500-1500 m2/g | As determined by nitrogen adsorption/desorption A cubic centimeter of an aerogel has about the same surface area as one soccer field) |
Solid percentage in volume | 0.13-15 % | Typically 5 % (95 % free space) |
Mean pore diameter | 20-150 nm | As determined by nitrogen adsorption/desorption (varies with density) |
Primary particle diameter | 2-5 nm | Determined by transmission electron microscopy |
Index of refraction | 1.007-1.24 | Very low for solid material (nair= 1.004) |
Thermal tolerance | Up to 500 C | Shrinkage begins slowly at 500 C, increases with increasing temperature. Melting point is ~1200ºC |
Poisson’s ratio | 0.2 | Independent of density, similar to dense silica. Determined using ultrasonic methods. |
Young’s modulus | 0.1-300 MPa | Very small (<104) compared to dense silica |
Tensile strength | 16 kPa | For density of 0.1 g/cm3 |
Fracture toughness | 0.8 kPa.m1/2 | For density of 0.1 g/cm3. Determined by 3-point bending |
Dielectric constant | 1.1 | For density of 0.1 g/cm3, very low for a solid material (kair= 1) |
Acoustic impedance | 104 Kg/m2.s | Determined using ultrasonic methods al KHz frequency. |
Sound velocity through the medium | 20-800m/s | 100 m/s for density of 0.07 g/cm3, one of the lowest velocities for a solid material |
Optical property | Transmittance>90% (630nm) | Transparent-blue haze |
Thermal conductivity | 0.02 W/mK (20C) | Very low thermal conductivity. 2 cm slab provides the same insulation as 30 panes of glass |
World Record Held by AEROGELS
Lowest density solid (0.0011 g cm-3)
Lowest optical index of refraction (1.002)
Lowest thermal conductivity (0.016 W m-1 K-1)
Lowest speed of sound through a material (70 m s-1)
Lowest dielectric constant from 3-40 GHz (1.008)
MANUFACTURING OF AEROGEL
A common way to synthesize gels at room temperature corresponds to a chemical reaction implying metal alkoxides and water in an alcoholic solvent. The majority of silica aerogels prepared utilizes silicon alkoxide precursors. The most common of these are tetramethyl orthosilicate (TMOS or Si(OCH3)4), and tetraethyl orthosilicate (TEOS or Si(OCH2CH3)4). However, many other alkoxides, containing various organic functional groups, can be used to impart different properties to the gel [5, 6]. The first reaction is a hydrolysis which induces the substitution of OR groups linked to silicon by silanol Si-OH groups. A condensation reaction occurs when two silanol groups (Si-OH + HO-Si) react together to form Si-O-Si (siloxane) bonds, which lead to the silica network formation.
The hydrolysis reaction replaces alkoxide groups (OR) with hydroxyl groups (OH). Subsequent condensation reactions involving the silanol groups produce siloxane bonds (Si¯O¯Si) plus the by-products alcohol (ROH) or water. Because water and alkoxysilanes are immiscible, a mutual solvent such as alcohol is normally used as a homogenizing agent. The final density of the aerogel depends on the concentration of silicon alkoxide monomers in the solution. The balanced chemical equation for the formation of a silica gel is:
Si(OR)4 (liq.) + 2H2O (liq.) = SiO2 (solid)+ 4HOR (liq.)
The stoichiometry of the reaction requires two moles of water per mole of alkoxysilane. In practice, this amount of water leads to incomplete reaction, and weak, cloudy aerogels. Therefore, most aerogel recipes use a higher water ratio than is required by the balanced equation (anywhere from 4-30 equivalents).
The gel point is the time at which the network of linked oxide particles spans the container holding the sol. At the gel point, the sol becomes a gel. This two-phase material, a solid part and a liquid part, consists of shaped solid exhibiting specific properties.
The solid part is formed by the three-dimensional network of linked oxide particles. The liquid part (the original solvent of the sol and a small amount of water) fills the free space surrounding the solid part. The liquid and solid parts of a gel occupy the same apparent volume.
As condensation reactions progress the gel will gain rigidity. At this point, the gel is usually removed from its mould. However, the gel must be kept covered by alcohol to prevent evaporation of the liquid contained in the pores of the gel. Evaporation causes severe damage to the gel and will lead to poor quality aerogels..
The balanced chemical equation for the formation of a silica gel from TEOS is:
Si(OCH2CH3)4 (liq.) + 2H2O (liq.) = SiO2 (solid) + 4HOCH2CH3 (liq.)
Catalysts
The kinetics of the above reaction is impractically slow at room temperature, often requiring several days to reach completion. For this reason, acid or base catalysts are added to the formulation. The amount and type of catalyst used play key roles in the microstructural, physical and optical properties of the final aerogel product .
Acid catalysts can be any protic acid, such as HCl. Base-catalysis usually uses ammonia, or ammonia buffered with ammonium fluoride. Aerogels prepared with acid catalysts often show more shrinkage during supercritical drying and may be less transparent than base catalyzed aerogels. The microstructural effects of various catalysts are harder to describe accurately, as the substructure of the primary particles of aerogels can be difficult to image with electron microscopy (section 3.7). All have small (2-5 nm diameter) particles that are generally spherical or egg-shaped. With acid catalysis, however, these particles may appear less defined than those in base-catalyzed gels.
Two-Step Aerogels
Typical acid or base catalyzed gels are often classified as "single-step" gels, referring to the "one-pot" nature of this reaction. A more recently developed approach uses pre-polymerized TEOS as the silica source. Pre-polymerized TEOS is prepared by heating an ethanol solution of TEOS with a sub-stoichiometric amount of water and an acid catalyst. The solvent is removed by distillation, leaving a viscous fluid containing higher molecular weight silicon alkoxy-oxides. In a second step, this material is redissolved in ethanol and reacted with additional water under basic conditions until gelation occurs. Gels prepared in this way are known as "two-step" acid-base catalyzed gels. Pre-polymerized TEOS is available commercially from Silbond Corp. (Silbond H-5).
These slightly different processing conditions impart important changes to the final aerogel product. Single-step base catalyzed aerogels are typically more brittle than two-step aerogels. Moreover, two-step aerogels have a smaller and narrower pore size distribution and are often optically clearer than single-step aerogels .
Aging and Soaking
At the gel point the silica backbone of the gel contains a significant number of unreacted alkoxide groups. Sufficient time must be given for the strengthening of the silica network. This can be enhanced by controlling the pH and water content of the covering solution. Common aging procedures for base catalyzed gels typically involve soaking the gel in an alcohol/water mixture of equal proportions to the original sol. The gels are soaked in this solution for up to 24 hours. This step, and all subsequent processing steps, is diffusion controlled. Diffusion is affected by the thickness of the gel. Then, the time required for each processing step increases dramatically as the thickness of the gel increases.
After aging the gel, all water still contained within its pores must be removed prior to drying. This is simply accomplished by soaking the gel in pure alcohol several times until all the water is removed. Again, the length of time required for this process is dependent on the thickness of the gel. Any water left in the gel will not be removed by supercritical drying, and will lead to an opaque, white, and dense aerogel.
Chapter II shows that variations in synthesis conditions (for example, ratio H2O/Si, the catalyst type and concentrations, the type of solvent, temperature and pressure of supercritical drying) cause modifications in the structure and properties of the obtained silica aerogels . Thus, porous structure of silica aerogels strongly depends on preparation and drying parameters .
DRYING PROCEDURE OF GELS
An aerogel results from a supercritical drying process. This is where the liquid within the gel is removed, leaving only the linked silica network. The difference between classical drying and supercritical drying is shown in Figure From point 1 to 5, (purple arrow) the liquid is depressurized isothermally (classical drying). Consequently, we can say that xerogels refer to gels dried at temperature close to room temperature and under atmospheric pressure. The supercritical drying is performed inside an autoclave, which allows to overpass the critical point (PC, TC) of the solvent, as shown in Figure (Path 1-2-3-4-5); or by prior solvent exchange with liquid CO2 followed by supercritical CO2 venting (lower temperature drying).
The supercritical fluid is a substance that is above its critical pressure and critical temperature; it possesses some properties in common with liquid (density, thermal conductivity) and some in common with gas (fills its container, does not have surface tension). A more detailed description of supercritical fluids can be found in Annex I). Strong inorganic solids are commonly dried using alcohol (or acetone) as solvent or dried using CO2 as solvent.
Under ambient conditions, during the evaporation of the solvent, a liquid-vapor interface is formed within the pores of the gel. The surface tension of the liquid creates a concave meniscus in each capillary. By evaporation, the meniscus recedes and the compressive force on the wall of the pores produces the collapse of the initial gel framework, or shrinkage (Fig). A liquid-vapor interface or the presence of a liquid in equilibrium with the vapor is only observed below the critical temperature and pressure of the solvent. Above the critical point (Fig)the liquid no longer existed. Supercritical fluid is a gaseous like-phase, so the liquid meniscus and its interfacial tension would not form in these conditions.
Using the high temperature drying procedure some problems may arise from the combination of high pressures and high temperatures (methanol critical parameters: Pc=81 bar, Tc=240C) , i.e. flammability of the solvents. Alternatively, supercritical drying with CO2 has been developed by substituting, under pressure, alcohol in the gel by liquid carbon dioxide and then drying the aerogel with carbon dioxide at supercritical conditions. CO2 is of particular interest due to its low critical temperature (31C), non-flammability, and non-toxicity. The process results in a reduction of the temperature and pressure required for drying aerogels.
CONCEPT OF SUPERCRITICAL DRYING
Supercritical drying (SCD) is a process by which the liquid in a substance is transformed into gas in the absence of surface tension and capillary stress and is the process most commonly used to transform gels into aerogels. Supercritical drying is performed to replace the liquid in a material with a gas isolate the solid component from the material without destroying the material’s delicate nanostructured pore network.
The Critical Point
All pure substances that do not otherwise decompose possess a critical point, a specific temperature and specific pressure characteristic of that substance at which its liquid and gaseous phases become indistinguishable.
Molecules/atoms in gases, liquids, and even solids are constantly in motion to varying degrees. As a substance is heated, the kinetic energy of the molecules of that substance increases and the molecules move around faster. In a liquid, the molecules are close enough together that they experience attractive (sticky) forces between one another. These attractive forces weakly bind the molecules together into little packs or chains but can be easily overcome as the molecules move around and vibrate. The degree to which molecules of a substance stick to each other is called its “surface tension”. The surface tension of water is what allows water skippers to walk on the surface of water. In a gas, however, the molecules are far apart and are not close enough to other molecules to experience attractive forces. Additionally, the molecules in a gas are moving so fast that when two molecules do collide, they pretty much just bounce off each other instead of sticking.
Equilibrium
A substance in its liquid phase is are always in equilibrium with a gaseous (or “vapor”) phase as well. For example, if you put water in a two-liter bottle and seal the bottle, the water will evaporate until a certain pressure of water vapor is reached above the liquid in the bottle. Once that pressure is reached, the number of molecules evaporating from the liquid is about the same as the number of molecules recondensing from the vapor into the liquid, a phenomenon which we call “equilibrium”. The pressure of water that will result is exclusively a function of the temperature of the liquid–the hotter the liquid, the higher the pressure. This is why it is generally more humid in the summer than in the winter (depending on how much water there is around where you live). Importantly, the pressure of the water is independent of the pressure of other gases over the water. For example, if you pressurized the bottle with two atmospheres of nitrogen gas, the pressure contributed from the water, its “partial pressure” would be the same, although now it would be a smaller percentage of the total pressure in the bottle since we’ve added more nitrogen.
So now that we recognize that the liquid phase of a substance is always in equilibrium with a vapor phase and that the partial pressure of the vapor phase is only a function of the temperature of the liquid, we can begin to understand the critical point.
PV=nRT
that is, the pressure of a gas times the volume of its container is equal to the number of moles of substance that make up the gas times the gas constant “R” times the temperature of the gas.
BUT what you might not remember is that this really only holds for low-pressure gases at reasonably high temperatures, in which the molecules are far apart and don’t stick to each other.
At higher pressures, and at temperatures close to where the vapor is about to recondense into a liquid, this equation doesn’t hold. And so scientists came up with other equations that work better for these ranges, such as the Van der Waals equation.
But at the critical point, even these more complicated equations fall apart.
Imagine we have a liquid in a sealed, rigid container that isn’t going to blow up if we pressurize it. As the liquid is heated, the pressure of its vapor will increase. As this happens, more and more molecules enter the vapor phase, making the vapor more and more crowded and making the average distance between two molecules smaller. Eventually, the molecules in the gas are so close together that they are basically as close as they would be in a liquid. At the same time, as the liquid is heated, the molecules in the liquid get more kinetic energy and start moving faster and faster until they are moving so fast that they scream past one another, overwhelming the weak attractive forces that hold the liquid together. The result is a liquid which can no longer hold together and gas so dense it is as dense as the liquid and the two phases merge into a single phase with the density of liquid that expands to fill its container like a gas. The specific temperature and pressure at which this happens is the “critical point” of the substance and the single merged semi-liquid/semi-gas phase is called a “supercritical fluid”.
Properties of Supercritical Fluids
Supercritical fluids are strange substances. Since they are dense like a liquid, they conduct heat like liquids (if you could touch a supercritical fluid, it would feel kind of like a liquid). But at the same time, supercritical fluids expand and compress like gases. Supercritical fluids often also possess the ability to dissolve stuff that their liquid version cannot. For example, supercritical carbon dioxide exhibits the uncanny ability to dissolve fluorinated hydrocarbons, like some types of Teflon® used in lubricants. Supercritical fluids are infinitely compressible at the critical point and as a result exhibit a significant density gradient due to the weight of the fluid pushing down on itself (they are denser at the bottom than they are at the top).
Surface Tension and Capillary Stress
When the liquid in a porous material such as a gel or a dead bug is removed by evaporation or boiling, capillary stress in the pores of the material cause the struts of the pore network to collapse and the material shrinks. Think of the liquid molecules in a pore as being a bunch of little magnets all stuck together and think of the pore as being made out of flimsy strips of sheet metal. Now imagine pulling on one of the magnets and all of the other magnets getting pulled up with it until you pluck the magnet out from the bunch. The pile then collapses back to fill in the gap from where you pulled out the magnet and pulls the flimsy sheet metal walls of the pores inwards. This is sort of what happens when a liquid molecule is evaporated from a pore–all of the other molecules get pulled in and tug on the struts that carve out the volume of the pore. The more molecules evaporate, the more the struts get tugged on. This is called “capillary stress”. If the liquid has a high surface tension, the molecules in the liquid are strongly attracted and the capillary stress exerted on the pores of the material will be higher.
Supercritical drying entails heating/pressurizing the liquid in a material past its critical point, at which it is transformed into a supercritical fluid. As a liquid approaches its critical point, the molecules in the liquid move past each other faster and faster and stick together less, meaning the surface tension of the liquid decreases. Thus the capillary stress that the liquid can exert decreases as well. Finally at the critical point the supercritical fluid loses all surface tension and can no longer exert capillary stress.
At this point, the supercritical fluid can be removed from the pores of the material by depressuriziing the fluid while keeping it above its critical temperature (isothermal depressurization). If the temperature of the fluid drops below the critical temperature, liquid will start to rain out of the fluid. As pressure is released from the vessel containing all of this, molecules are removed from the fluid to the surroundings as a gas and the fluid becomes less dense. After a while, enough of the fluid will have been removed from the pressure vessel so that when it is cooled below its critical point there just isn’t enough substance to recondense to a liquid (the fluid is too low in density) and instead reverts to a gas.
Technically, any substance above its critical temperature and critical pressure is a supercritical fluid, but if it is much hotter than its critical temperature, the fluid really just behaves like a gas. So actually, only within a range of temperature and pressure near the critical point is the term supercritical fluid useful.
Supercritical Drying of Aerogels
A gel is prepared using sol-gel chemistry. The gel contains a mixture of organic solvent and water in its pores.
The gel is soaked in a pure organic solvent several times over the course of several days to remove the water from its pores.
Finally, the gel is supercritically dried in a pressure vessel one of two ways:
The gel is placed in a pressure vessel filled about half-way with the same liquid held in its pores (so if your gel has ethanol in its pores you would fill the pressure vessel with ethanol). The vessel is then sealed, heated past its critical temperature and pressure, and then isothermally depressurized to give aerogel.
The gel is placed in a pressure vessel and the pressure vessel is filled with liquid carbon dioxide. The liquid carbon dioxide is essentially another solvent that can displace the liquid in the pores in the gel. The gel is soaked in liquid carbon dioxide over the course of several days, flushing new carbon dioxide through every 12-24 h to replace the solvent in the pores of the gel with liquid carbon dioxide. After the solvent in the pores of the gel has been replaced by liquid carbon dioxide, the carbon dioxide is heated past its critical temperature and pressure. The vessel is then isothermally depressurized to give aerogel.
High-Temperature Supercritical Drying
Most organic solvents have relatively high critical temperatures of 300-600°C with critical pressures of 50-100 atm, and are dangerously flammable and potentially explosive at these conditions. As a result, high-temperature supercritical drying needs special safety precautions.
At its critical point, methanol can react with hydroxyl groups on the surface of the gel’s solid framework to form methoxy groups. These methoxy groups end up making the aerogels partially hydrophobic (water-resistant) and is the reason why high-temperature supercritically dried silica aerogels are generally higher quality.High-temperature supercritical drying from organic solvents is also the best way to minimize shrinkage of the gel, allowing for supercritical drying of much lower-density gels than from carbon dioxide.
Certain aerogels, such as organic polymer aerogels, can’t be made using high-temperature supercritical drying because they decompose or react at the temperatures at which most organic solvents are supercritical.
Low-Temperature Supercritical Drying from Carbon Dioxide (the Hunt Process)
Instead of using flammable, explosive solvents, a safer, non-flammable solvent can be used instead–carbon dioxide. In this process, the organic solvent in a gel is replaced with liquid carbon dioxide by soaking. The liquid carbon dioxide can supercritically extracted at a much lower temperature (31.1°C) than an organic solvent and without the risk of combustion.
Certain aerogels can’t be made with liquid carbon dioxide, such as certain metal oxides, because they react with carbon dioxide to form metal carbonates.
When the original solvent in the gel is exchanged for liquid carbon dioxide, the gel may shrink slightly. This is caused by favorable interactions between the two liquids resulting in a mixture with molecules closer together than molecules in either of the liquids separate. This is called ΔV of mixing. Solvents with low ΔV’s of mixing with liquid carbon dioxide include acetone and amyl acetate, although ethanol and methanol are pretty good. Importantly, it is the solvent exchange into liquid carbon dioxide, which has a relatively low density of 0.59 g cm-3 that causes the gel to shrink, no the supercritical drying process. In general, aerogels made using supercritical drying from carbon dioxide may shrink up to 5%
AEROGEL APPLICATIONS
A nearly transparent, very lightweight material that is a dry gel principally made from silica (silicon dioxide) and 96% air. Dubbed a "Super Material", aerogel is the world's lightest solid, weighing as little as three times that of air and exhibiting superb insulating properties. Although aerogel looks like it could float away, it has very high compression strength. Theoretically, a block weighing less than a pound could support a weight of half a ton. Aerogels real strength is its incredible insulating effects on any kind of energy transfer; thermal, electrical or acoustic. Aerogel can damp out almost any kind of energy. A one-inch thick Aerogel window has the same insulation value as 15 panes of glass and trapped air - which means a conventional window would have to be ten-inches thick to equal a one-inch thick aerogel window.The following applications for aerogels are associated with certain properties of aerogel materials. In many cases, the application is associated with a single property even if the aerogels have a combination of properties appropriate to the given application.
THERMAL PROPERTIES
Aerogels are good thermal insulators because they almost nullify the three methods of heat transfer (convection, conduction, and radiation). They are good conductive insulators because they are composed almost entirely from a gas, and gases are very poor heat conductors. Silica aerogel is especially good because silica is also a poor conductor of heat (a metallic aerogel, on the other hand, would be less effective). They are good convective inhibitors because air cannot circulate through the lattice. Carbon aerogel is a good radiative insulator because carbon absorbs the infrared radiation that transfers heat at standard temperatures. The most insulative aerogel is silica aerogel with carbon added to it.
POROSITY AND SURFACE AREA APPLICATIONS
Due to their high porosity, their very large inner surface area (easily accessible because of the open porosity), and the controllable dispersion of the active component, they are especially active catalysts or catalytic substrates . There are numerous references of this application for various aerogels and doped aerogels .
Moreover, the high porosity and large surface areas lead to applications as filters , absorbing media for desiccation , filters, reinforcement agents, pigments, gellifying agents , waste containment , encapsulation media , and pesticides.
Electrodes in capacitors
The carbon aerogels have been used as electrodes capacitors in energy storage devices known as double layer capacitor because they are electrically conductive with a very large surface area . The stored energy in these devices can be released faster than conventional batteries with high power densities. Thus, have potential application in electric vehicles, microelectronics, and hydrogen fuel storage
Capacitive deionization
One of the promising new applications for aerogels is in a cost-effective purification process. The carbon aerogel capacitive deionization process works by sending solutions with various positively and negatively charged ions through an electrochemical cell consisting of numerous electrodes containing carbon aerogels in the form of sheets. The aerogel process can have a variety of uses ranging from extracting harmful contaminants from industrial waste water to desalinizing seawater.
OPTICAL PROPERTY APPLICATIONS
Aerogel is transparent when its microstructural components are very small compared with the wavelength of light. Transparent aerogels, together with their exceptional thermal insulation ability, have been considered for use as super-insulating sheets in double walled window systems because help considerably to reduce thermal losses in windows .
Translucent aerogels have been proposed to improve the efficiency of solar thermal energy storage devices . Moreover, the ultra-low density aerogels can be used as lightweight mirror backings . Aerogels have been used to prepare ultra-pure, full-density silica glass by sintering at temperatures below the melting temperature of silica . Silica aerogels with silicon exhibits strong photo-luminescence (luminescence stimulated by visible or ultraviolet radiation). Silica aerogel, doped with radioactive tritium and phosphor, makes an efficient radio-luminescent light source. There is also evidence for quantum confinement in nanoparticle-loaded silica aerogels for producing blue light emission
ACOUSTICAL AND MECHANICAL APPLICATIONS
Aerogels may also have acoustic and mechanical applications. Because of their unusual structure, aerogels have low sound velocities, as low as 30 meters per second.
Another important acoustic property of aerogel is its mechanical impedance. The impedance is the product of density and the sound velocity of the material. Since both are low, silica aerogel has the lowest impedance of all solid material. This allows the aerogels to be used for coupling sound waves in air to a transducer (device that converts energy from one form to another), this may be useful either to generating or detecting sound. Therefore, they should be efficient ultrasonic devices as acoustic impedance matching , and sound absorption (anechoic chambers)
Shock compression experiments
Aerogels have also been proposed as a shock absorbing material. One of the earliest experiments was to measure shock compression in silica aerogels . The low density of the silica aerogel allowed more internal energy could be deposited in it.
ELECTRICAL AND ELECTRONIC APPLICATIONS
Silica aerogel is an electrical insulator with a low dielectric constant, k (k is the measure of the ability of a material to store electrical potential energy under the influence of an electric field). The velocity of signal propagation in a chip is dependent on the dielectric constant of the surrounding electrical insulation. The lower the dielectric constant, the higher the velocity. Therefore, thin aerogel films are almost ideal dielectrics for ultra-fast integrated circuits.
The bulk aerogels can be used for microwave electronics and high voltage insulators . The pure carbon aerogels are quite electrically conductive, so they have applications as electrodes for batteries, fuel cells, and capacitors . Other metal oxide aerogels have been made, which exhibit super-conducting behavior, thermoelectric behavior , and piezoelectric properties
SPACE APPLICATIONS
Aerogels have already captured cosmic dust while on the European Retrieval Carrier (EURECA) satellite and in Space Shuttle experiments , and will capture cometary‟s dust in NASA's STARDUST project. Lightweight silica aerogels have also been proposed as a contaminant collector, to protect space mirrors from volatile organics .
MARS Rover
Aerogels were used to insulate the Mars Rover, where its lightness and strength were established as ideal. That's the primary reason aerogel was used as insulation on the Sojourner Mars rover in 1997. As night fell on Mars, the temperature dropped down to -67 C (-88 F). Although the temperature outside was colder than Antarctica in winter, it remained a balmy 21 C (70 F) inside the Rover, where sensitive electronics were protected from the hard freeze.
Aerogel sample collectors
Comet and interstellar particles are collected in ultra low density aerogel. More than 1,000 square centimeters of collection area is provided for each type of particle (cometary and interstellar). The collector tray contains ninety blocks of aerogel in a metal grid. The appearance of the grid has been likened to an ice cube tray; the round collector is about the size of a tennis racket.When the spacecraft flew past the comet, the impact velocity of the particles in the coma as they were captured was 6100 metres per second, up to nine times the speed of a bullet fired from a rifle. Although the captured particles were each smaller than a grain of sand, high-speed capture could have altered their shape and chemical composition — or vaporized them entirely.
Stardust capsule with aerogel collector deployed
To collect the particles without damaging them, a silicon-based solid with a porous, sponge-like structure is used in which 99.8 percent of the volume is empty space. Aerogel is 1,000 times less dense than glass, another silicon-based solid. When a particle hits the aerogel, it buries itself in the material, creating a carrot-shaped track up to 200 times its own length, as it slows down and comes to a stop — like an airplane setting down on a runway and braking to reduce its speed gradually. Since aerogel is mostly transparent — a property earning it the nickname "solid smoke" or "blue smoke" — scientists will use these tracks to find the tiny particles.
The aerogel was packed in a Sample Return Capsule (SRC) which was released from the spacecraft just before reentry, for a separate landing on a parachute, while the rest of the spacecraft fired its engines, putting it into orbit around the sun.
CONCLUSION
Aerogel will probably be a common household name. Although it is not yet ready for commercial use, Americas Fortune magazine's "Technology to Watch" column mentioned 800 potential products that could be manufactured out of aerogel, citing everything from surfboards to satellites. With the use of aerogel in the Sojourner Mars rover, in the StarDust spacecraft, and in the hundreds of other possible products and applications, the "unobtainium" once only dreamed about may finally be just around the corner.
FUTURE
Today significant efforts are underway to further mechanically strong aerogels, aerogels of new compositions for sensors and energy production, and to apply aerogels for use as hydrogen storage media. Metal aerogels are just around the corner. More advanced supercapacitors that rival today’s batteries are becoming likely. Hydrogen production using cleverly-engineered semiconductor aerogels will change the way we think about energy and fuel. Smart materials made possible by the unique combinations of materials properties exhibited by aerogels will enhance and impact our daily lives.
GLOSSARY
Hydrolysis: The reaction of a metal alkoxide (M-OR) with water, forming a metal hydroxide (M-OH).
Condensation: A condensation reaction occurs when two metal hydroxides (M-OH + HO-M) combine to give a metal oxide species (M-O-M). The reaction forms one water molecule.
Sol: A solution of various reactants that are undergoing hydrolysis and condensation reactions. The molecular weight of the oxide species produced continuously increases. As these species grow, they may begin to link together in a three-dimensional network.
Gel Point: The point in time at which the network of linked oxide particles spans the container holding the Sol. At the gel point the Sol becomes an Alcogel.
Precursors: In the sol-gel process, the precursors (starting compounds) for preparation of a colloid consist of a metal or metalloid element surrounded by various ligands (appendages not including another metal or metalloid atom). The latter is an example of an alkoxide, the class of precursors most widely used in sol-gel research.
Shrinkage: Shrinkage of a gel, either during syneresis or as liquid evaporates during drying, involves deformation of the network and transport of liquid through the pores.
Aerosol: A colloidal suspension of particles in a gas (the suspension may be called a fog if the particles are liquid and a smoke if the are solid).
Aging :The term aging is applied to the process of change in structure and properties after gelation. Bond formation does not stop at the gel point. In the first place, the network is initially compliant, so segments of the gel network can still move close enough together to allow further condensation (or other bond-forming processes). Moreover, there is still a sol within the gel network, and then those smaller polymers or particles continue to attach themselves to the network.
Colloid :A mixture in which one substance is divided into minute particles (called colloidal particles) and dispersed throughout a second substance. The dispersed phase is so small (1–1000 nm) that gravitational forces are negligible and interactions are dominated by short-range forces, such as van der Waals attraction and surface charges. The mixture is also called a colloidal system, colloidal solution, or colloidal dispersion. Familiar colloids include fog, smoke, and homogenized milk.
Emulsion: Is a suspension of liquid droplets in another liquid. These types of colloids can be used to generate polymers or particles from which ceramic materials can be made
Gel :A gel consists of two parts, a solid part and a liquid part. The solid part is formed by the three-dimensional network of linked oxide particles. The liquid part (the original solvent of the sol) fills the free space surrounding the solid part. The liquid and solid parts of a gel occupy the same apparent volume. Thus, a gel is a substance that contains a continuous solid skeleton enclosing a continuous liquid phase. The gel can be removed from its original container and can stand on its own. The continuity solid structure gives elasticity to the gel (as in the familiar gelatin dessert).
Alcogel (wet gel): At the gel point, the mixture forms a rigid substance called an alcogel. The alcogel can be removed from its original container and can stand on its own. An alcogel consists of two parts, a solid part and a liquid part. The solid part is formed by the three-dimensional network of linked oxide particles. The liquid part (the original solvent of the Sol) fills the free space surrounding the solid part. The liquid and solid parts of an alcogel occupy the same apparent volume.
Supercritical fluid: A substance that is above its critical pressure and critical temperature. A supercritical fluid possesses some properties in common with liquids (density, thermal conductivity) and some in common with gases (fills its container, does not have surface tension).
Aerogel: What remains when the liquid part of an alcogel is removed without damaging the solid part (most often achieved by supercritical extraction). If made correctly, the aerogel retains the original shape of the alcogel and at least 50% (typically >85%) of the alcogel's volume.
Xerogel: What remains when the liquid part of an alcogel is removed by evaporation, or similar methods. Xerogels may retain their original shape, but often crack. The shrinkage during drying is often extreme (~90%) for xerogels.
Dinesh Kumar, dany12ac@gmail.com,Mechanical ENGG.
ReplyDeleteI need this report and ppt for my college seminar. Kindly help me.
bhosle dhiraj
ReplyDeletebhosaledhiraj90@gmail.com
i need the seminar on aerogel plz sent to my mail given above