
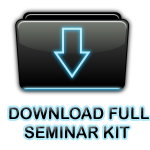
In the commercial realm, fog-bound airports could be eliminated as a cause for flight delays or diversions. For security concerns, imaging of concealed weapons could be accomplished in a nonintrusive manner with PMMW imaging. Like IR and visible sensors, a camera based on PMMW sensors generates easily interpretable imagery in a fully covert manner; no discernible radiation is emitted, unlike radar and lidar. However, like radar, PMMW sensors provide penetrability through a variety of low-visibility conditions (moderate/heavy rainfall is an exception). In addition, the underlying phenomenology that governs the formation of PMMW images leads to two important features. First, the signature of metallic objects is very different from natural and other backgrounds. Second, the clutter variability is much less in PMMW images than in other sensor images. Both of these characteristics lead to much easier automated target detection with fewer false alarms.
The wide range of military imaging missions that would benefit from an imaging capability through low-visibility conditions, coupled with its inherent covertness, includes surveillance, precision targeting, navigation, aircraft landing, refueling in clouds, search and rescue, metal detection in a cluttered environment, and harbor navigation/surveillance in fog. Similarly, a number of civilian missions would benefit, such as commercial aircraft landing aid in fog, airport operations in fog, harbor surveillance, highway traffic monitoring in fog, and concealed weapons detection in airports and other locations. This article introduces the concept of PMMW imaging, describes the phenomenology that defines its performance, explains the technology advances that have made these systems a reality, and presents some of the missions in which these sensors can be used.
2. OVERVIEW OF MILLIMETER WAVE RADIOMETRY
The regime of the electromagnetic spectrum where it is possible for humans to see is that part where the sun’s radiance peaks (at about 6,000 K): the visible regime. In that regime, the human eye responds to different wavelengths of scattered light by seeing different colors. In the absence of sunlight, however, the natural emissions from Earth objects (at about 300 K) are concentrated in the IR regime. Advances in IR-sensor technology in the last 30 years have produced detectors sensitive in that frequency regime, making night vision possible. The exploitation of the millimeter-wave regime (defined to lie between 30 and 300 GHz, with corresponding wavelengths between 10 and 1 mm) follows as a natural progression in the quest to expand our vision. The great advantage of millimeter-wave radiation is that it can be used not only in day and night conditions, but also in fog and other poor visibility conditions that normally limit the “seeing” ability of both visual and IR sensors.
3. ATMOSPHERIC PROPAGATION
The usefulness of millimeter waves lies in the peculiarities of atmospheric attenuation phenomenology’s over the prescribed frequency range. Figure 1 shows the attenuation of electromagnetic signals in decibels per kilometer of the propagation path length from the microwave through the visible regime [4]. This spans the frequency range from 10 GHz to 1,000 THz, with corresponding free-space wavelengths from 3 cm to 0.3 µm.
Propagation of electromagnetic waves over this frequency range is subject to continuum and resonant absorption by various atmospheric constituents, including water in vapor and droplet form, oxygen, nitrogen, carbon dioxide, ozone, etc. In clear, dry weather, IR and visible radiation propagate with little attenuation; however, atmospheric water content in the form of fog, clouds, and rain causes significant absorption and scattering. In the far IR and sub millimeter-wave regime, significant attenuation occurs from water vapor. Conversely, in the millimeter-wave regime, there are propagation windows at 35, 94, 140, and 220 GHz, where the attenuation is relatively modest in both clear air and fog. Even taking into account the much higher blackbody radiation at IR and visible frequencies, millimeter waves give the strongest signals in fog when propagated over distances of useful interest (see Figure 2). In fact, millimeter-wave radiation is attenuated millions of times less in clouds, fog, smoke, snow, and sandstorms than visual or IR radiation. This is the critical advantage of PMMW imagery. Moreover, millimeter-wave imagery is minimally affected by sun or artificial illumination. Therefore, it operates equivalently in clear or low-visibility conditions during the day or night. It is this ability that makes millimeter waves the best candidate for imaging in most conditions of low visibility.
4. EMISSIVITY AND BRIGHTNESS TEMPERATURE
Objects reflect and emit radiation in the millimeter- wave regime, just as they do in the IR and visible regimes. The degree to which an object reflects or emits is characterized by the emissivity (e) of the object. A perfect radiator (absorber) has e =1 and is known as a blackbody. A perfect reflector (non absorber) has e = 0.
The emissivity of an object (which is polarization dependent) is a function of the dielectric properties of its constituents, its surface roughness, and the angle of observation. A sampling of emissivities for diverse materials at various frequencies, at normal incidence, and measured at one polarization, is given in Table 1 (based on work performed at NGST.) When an object’s physical thermodynamic temperature To is multiplied by its emissivity e, the resultant product is defined as the object’s surface brightness (or radiometric) temperature TS.
Ts = e * To (1)
The variation in emissivities of common scene materials, as shown in Table 1, is an important factor in the generation of scene images by causing variations in the power radiated from different parts of the scene. If this were the only factor involved, then images could be formed by simply mapping measured values of TS in the scene. However, the way the scene is illuminated is critical in the way the scene actually looks. For example, a metal plate with e = 0 will have TS = 0, but, because it is highly reflective, it will appear to have the radiometric temperature of whatever it is reflecting. This effect is captured by a surface scattered radiometric temperature TSC defined as the product of the objects reflectivity ñ and the radiometric temperature Tilluminator of whatever happens to be illuminating it.
Tsc = p* Tilluminator (2)
Combining the terms TS and TSC gives the effective radiometric temperature TE of the object.
TE = Te + Tsc (3)
In outdoor imaging, the primary source of illumination is the down-welling of radiation from the sky itself. When a radiometer (an instrument for detecting thermal radiation) is aimed at the zenith, the radiometer will detect not only the residual radiation from deep space, but the low level of radiation that down-wells from the atmosphere itself, yielding a measured brightness temperature on the order of only ~60 K at 94 GHz . Thus, a metal object with e = 0 and p =1 will have TE ~ 60 K, and will, thus, appear very cold in an image.
Imaging can be accomplished by measuring TE as a function of position in the scene, thus creating a two-dimensional (2-D) map or image of the scene. Note that the above is highly simplified, not taking into account such factors as viewing angles, surface orientation, polarization effects, variations in the sky temperature as a function of angle relative to zenith, and both atmospheric emissions and attenuation along the line of sight of the measurement.
5. IMAGING PRINCIPLES
The observed radiometric temperature of a scene is based on the following factors: emissions from scene constituents, reflections of the down-welling sky radiation by the scene, up-welling atmospheric emissions between the scene and the observer, and propagation of electromagnetic energy from the scene to the observer.
Figure 3 illustrates these scene constituents. Image contrast is provided by differences in material, temperature, and sky illumination of the scene. Sky illumination is typically low level, i.e., radiometrically cold, at the zenith, rising to ambient temperatures at the horizon. Notice that the sun’s illumination is not needed to generate a millimeter-wave radiometric temperature-based image of the scene.
An example of a radiometric image is illustrated in Figure 4. This figure contains a series of images that were obtained with a single 94-GHz radiometer mounted at the focus of a scanning 48-in diameter Cassegrain dish antenna.
These images, acquired in both clear (b) and foggy (near zero visibility) weather conditions (d), are of a runway as it would look to a pilot just before touchdown. Visible images (a) and (c) are included for comparison. The scanning process involved the repeated tilting of the dish antenna aim point up and down while slowly panning the entire dish assembly from left to right. In this manner, the footprint of the antenna pattern on the scene was slowly moved over the entire scene. In this radiometric (or PMMW) image, the increasingly darker shades denote increasingly colder radiometric temperatures, although this can be reversed for aesthetic or other reasons.
The equipment parked next to the runway appears cold because their metal surfaces, which are nearly fully reflective, reflect the cold overhead sky. The asphalt runway, a good reflector at grazing incidence, reflects the sky at the horizon, which is warmer than the overhead sky. The dirt adjacent to the runway is colder than the asphalt because the roughness of the dirt surface mixes the reflections from various parts of the sky, effectively lowering the dirt’s radiometric temperature.
The important features to notice in Figure 4 are the images through fog. The visual images are totally obscured except for the near foreground, while the PMMW images are nearly unaffected by the fog. An interesting feature that emerges from the PMMW images is the mirror reflection of the parked equipment on the asphalt runway. This occurs because the asphalt runway, instead of reflecting the warm sky at the horizon, now sees a colder part of sky overhead via reflections off the equipment. Note that PMMW images , unlike radar images, have a visual quality, but with less resolution than a visible light picture.
The limitation of PMMW imaging is that image resolution, i.e., sharpness, is degraded compared to visible and IR images. The lower resolution is a consequence of the longer wavelengths used relative to the aperture size of the sensor’s collection optics. Resolution improves with increases in the aperture size. This is illustrated in Figure 5 at 94 GHz (3.1 mm wavelength) for three different dish antenna aperture sizes. As can be seen, the image becomes sharper as the aperture diameter increases. The same effect can also be attained with a higher frequency (shorter wavelength).
The limits on the resolution, however, will always limit the range over which imaging can be performed, and can be more restrictive than the atmospheric propagation constraints. For example, In Figure 5(b), just to the right of the large black bar in the upper left of the image, a large metal hangar building, are three black spots. These are school buses at a range of 3,570 ft.
These spots blur out to near nonexistence in Figure 5(d). Thus, the challenge is to construct a sensor that has a high enough frequency that is small enough to fit easily within an aircraft platform, for example, and yet still provide sufficient resolution to permit safe and accurate navigation, landing, surveillance, or other desired functions.
6. PMMW IMAGING
As previously stated, all objects radiate or reflect natural radiation across the frequency spectrum. Similar to a visible camera (which detects visible radiation) and an IR camera (which detects IR radiation), a PMMW camera detects millimeter-wave radiation from the scene. All cameras are composed of a means to measure the radiation level from the scene and a means for forming the image (directing the radiation onto the measurement means).
The following will briefly describe these means for the millimeter-wave regime, and will, for the most part, be recognized as extensions of what is done in the microwave regime. The measurement of the scene radiation can be performed by a variety of methods. A typical method utilizes radiometers, which are basically calibrated receivers of thermal radiation. Radiometers come in several configurations, but the two most prevalent are the total power and the Dicke radiometers . As the name implies, the total power radiometer simply integrates the power the receiver collects and provides a measurement of the total power collected. Likewise, the Dicke radiometer collects the scene radiation, but for only half the time. During the other half, it views a reference load, and by rapidly switching between the two sources of radiation, it obtains a measurement of the received power level that is less sensitive to instrumental drifts. The receiver itself, whether configured as a total power or Dicke radiometer, can be built in many ways.
The simplest is the bolometer, in which the incident radiation is focused onto a detector element, causing a temperature rise that is used to infer the incident power level. Somewhat more complex are electronic means that directly amplify the incident radiation and measure the power content of the resultant signal using a square-law detector. Finally, there are receiver designs that utilize both the amplitude and phase information of the incoming radiation. This seminar will deal primarily with total power or Dicke radiometer configurations using electronic amplification of the incoming signal. There are also many means for forming the image. As described earlier, a single focused receiver can be raster scanned across the scene to form the image. The manner in which the radiation is focused onto the receiver varies from system to system, ranging from dish antennas as used by radio astronomers, to the quasi-optical use of plastic lenses having the proper dielectric constants.
Systems have also been designed and built to scan a small array of focused receivers using mechanical methods (spinning mirrors or antennas, rotating arrays, etc.) Others scan a line array of focused receivers through the simple motion of an aircraft, a so-called “pushbroom” configuration in which the footprint of the array of receivers on the ground is swept along by the aircraft movement. Such a pushbroom camera was built by NGST for the Office of Naval Research and was used to create the 180° × 30° panoramic picture in Figure 6. Here the line array was mounted vertically and the camera was rotated about a vertical axis to form the image. The most sophisticated methods involve the electronic scanning of the antenna pattern across the scene through phased-array techniques and interferometric methods using sparse arrays of widely separated antennas as performed in radio astronomy and other surveillance systems. Research groups around the world in academia, industry, and government have utilized these and other very novel and innovative methods to form PMMW images
. In order to generate a video-like, real-time sequence of PMMW images, several methods have been investigated and developed around the world. These range from the rapid mechanical scanning of a small array, which has been shown to yield video-like PMMW imagery, to the complex but innovative phased-array approach utilizing the frequency regime to perform the required rapid scanning .A third approach uses a configuration similar to what is commonly used in visible and IR video cameras, that is, the use of a 2-D array of detectors. NGST has taken this approach in its development of PMMW imaging technology, taking advantage of the receiver technology it has developed, basically a complete radiometer on a chip.
As depicted in the sketch of Figure 7, the millimeter- wave radiation from the objects within a scene is focused onto a focal-plane array (FPA) of millimeter- wave receivers. By measuring and recording the variation in the resultant detected power or intensity levels across the scene, sequences of scene images can be collected and displayed to form a video-rate image stream.
7. PMMW CAMERA
Several recent technological advances have enabled the exploitation of millimeter waves. The advent of millimeter-wave monolithic integrated circuit (MMIC) technology has greatly increased the regime’s potential; direct, low-noise amplification, and detection of millimeter-wave radiation are now a reality. Low-noise amplifiers utilizing pseudomorphic high electron mobility transistor (PHEMT) technology on gallium arsenide (GaAs) substrates were first developed, then later combined with on-chip switches and a sensitive detector diode, to form a complete MMIC-based, 89-GHz Dicke-like receiver on a single 2 × 7-mm chip having a noise figure of ~5.5 dB and bandwidth of 10 GHz . The combination of high-sensitivity, low-cost, and low-power operation afforded by this MMIC device has enabled the construction of the world’s first FPA PMMW video camera, shown in Figure 8 .
The MMIC chip is shown in the upper left, and four of them are placed on a 1 × 4 module to form a building-block unit for the camera FPA. The full FPA contains 260 of these 1 × 4 Modules (1,040 receivers), arranged in 26 planar cards of 40 receivers each. It operates according to the concept outlined in Figure 7.
The PMMW video camera shown in Figure 8 uses an 18-in diameter plastic lens to collect and focus the radiation (a secondary lens within the camera is not visible), yielding a diffraction-limited 0.5° angular resolution. The size of the array, coupled with the optics, yields a 15° horizontal× 10° vertical field of view. A45° turningmirror directs the focused radiation onto the FPA. This mirror also serves to dither the image on the FPA, i.e., it moves the image to four locations at the corners of a 0.5 × 0.5-pixel square, thereby quadrupling the number of points and attaining Nyquist sampling of the scene. The camera is capable of an image update rate of 17 Hz (with a display rate of 30 Hz), with a minimum resolvable temperature of ~2 K.
The PMMW video camera was successfully tested on the ground, in flight, and at sea. Flight testing began in September 1997 aboard a U.S. Air Force KC-135 that was flown into numerous airports for runway approach imaging. Under contract with the Office of Naval Research, the PMMW video camera was also flown on a UH-1N helicopter and a Twin Otter aircraft along the coast between Los Angeles and San Diego, California. Video-rate image sequences were collected from altitudes ranging from 500 to 5,000 ft in both clear and overcast conditions.
8. APPLICATIONS
As clearly indicated in the following, PMMW imaging sensors are useful for a wide variety of commercial and military missions requiring near-all-weather operations, as well as covertness (since there are no camera emissions). In addition, high sensitivity to metal objects, man-made targets, and ship wakes, as well as reduced sensitivity to variations in clutter, make a PMMW video camera an effective sensor asset. All of the images were taken either with the NGST PMMW video camera (single frames or sequences of single frames from the video sequence can only be shown here) or with a single raster-scanned 94-GHz radiometer mounted on a dish antenna.
Aircraft Landing and Guidance
Covert, autonomous landing and taxiing in fog or smoke by helicopters and other aircraft is a mission of major relevance to both military and commercial operations. Figure 9 shows a sequence of images (6-s intervals, or every hundredth frame) taken from a PMMW video showing an approach to a dirt-over-dirt runway in the California desert in February 1999. The sequence starts at a distance of 1.7 nm from the runway threshold. The mountain range is approximately 12 nm away. The pilot relied only on this view, shown on a heads-up display along with an altimeter readout, and was able to fly the length of the runway at an altitude of 50 ft. Note that white is colder in these images. The smoother runway reflects the sky at the horizon, thus appearing warmer.
Low-Visibility Navigation and Situational Awareness
A PMMW imaging system can be employed on patrol boats and amphibious landing vehicles (LCACs) as well as other ground and seagoing vehicles to provide covert, near-all-weather visibility for navigation, target location, and other enhanced vision needs, especially in fog and through marine cloud layers. Figure 10 shows a raster-scanned image (using a single 94-GHz radiometer at the focus of a 48-in aperture dish antenna) of a harbor scene in Long Beach, California, one that would not have been impeded by fog if it had been present. Note the metal-domed structure reflecting the cold sky.
Reconnaissance and Surveillance
An aircraft or unmanned aerial vehicle equipped with a PMMW camera can be used for near-all weather look-down reconnaissance missions like the detection of ship wakes, low-radar cross-section boats, critical mobile, or other targets.
In Figure 12, a single frame from a sequence taken by the PMMW video camera mounted on a Twin Otter, is superimposed on the corresponding visible-light still from a cobore sighted video camera. In Figure 12(a), a C-17 is resolved from an altitude of 3,000 ft. The PMMW signature of metal targets is unique in the PMMW frequency regime, thus permitting auto-detection of such targets, as is evident in Figure 12, where black shows colder objects. The image on the right is of roadways and buildings imaged through a cloud bank, from an altitude of 1,900 ft.
Ground Navigation
Armored vehicles moving in fog, smoke, or sandstorms can benefit from a camera that presents a forward view essentially unaffected by those conditions, as an example but with no obscurant.
Figure 14 shows two automobiles imaged at ground level using a single 94-GHz radiometer mounted on a scanning 24-in dish antenna and adjusted to focus at approximately 50 ft...
Oil-Spill Detection
Oil on water will produce a detectable change in the observed radiometric temperature in the millimeter-wave regime through an interference effect similar to the way antireflection coatings on lenses work. In the case of millimeter waves, the oil layer can increase or reduce the radiometric temperature of the areas where the oil patches are by varying the effective emissivity of those areas through changes in thickness. The observed temperature can, in fact, be used to infer the thickness of the oil. The ability to detect oil on water using PMMW imaging techniques was demonstrated in 1990 during an oil spill off of Huntington Beach, California.
A single scanning 94-GHz radiometer was flown over the spill in a helicopter and generated the image shown in Figure 17. The scanning was done with a spinning mirror (the vertical axis of the image starts at 10° from nadir and goes upwards ~60°. The PMMW image displays radiometrically warm areas as white, and these areas correspond to the oil on the water surface. The dark background corresponds to open water that is clear of oil. Distortions inherent between the scanned image and the montage of visible wide-angle lens pictures have not been corrected.
Imaging of People and Concealed Weapons Detection
The imaging of people in the millimeter-wave regime produces images that are somewhat eerie, especially when done outdoors. Millimeter waves can easily penetrate clothing and hair. An image of a person will reveal a mannequin-like figure. The detected millimeter wave radiation is a combination of the natural emissions from the body and, since skin has a reflectivity on the order of 10%, what is reflected from the surroundings.
When outdoors, the sky is reflected off the skin of the upper surfaces of various body structures, while the warm ground is reflected from the lower body surfaces. This is illustrated in Figure 18, which was generated with a single 94GHz radiometer mounted on a scanning 24-in dish antenna adjusted to focus~25ft
If an object is placed under the clothing that has a dielectric constant different than skin, the millimeter wave image will reveal a radiometric temperature change caused by the object. For example, a metal gun hidden under the clothing will not only block the natural emissions from the body but will reflect the radiation from the surroundings. Even nonmetallic objects, such as plastics and ceramics, will have a similar effect. If indoors, where the room is illuminating the body with radiation at ~295 K, the body’s warmer emissions will be blocked or attenuated, and the object will be revealed to a PMMW imaging camera.
9. CONCLUSION
PMMW imaging has a variety of applications that can be of great use to make life safer and provide the military with a key advantage under low-visibility conditions, such as in fog, clouds, smoke, or sandstorms. Some of these applications have been presented with sample imagery, but many others are being studied by investigators around the world. Particularly exciting has been the advancement of MMIC technology to allow the development of the video-rate PMMW camera, and some of the vast amount of imagery test data has been presented here as well.
The near-all-weather operation holds the promise of providing an autonomous enhanced vision landing capability for commercial airlines, and the covert manner and the uniqueness of the PMMW signatures of targets of interest make this new window in the electromagnetic spectrum an extremely promising one for all branches of the military. With the advent of the PMMW video camera and the ability to view the world in the millimeter-wave regime, low-visibility conditions as a threat to safety or a hindrance to travel will be overcome.
No comments:
Post a Comment
leave your opinion