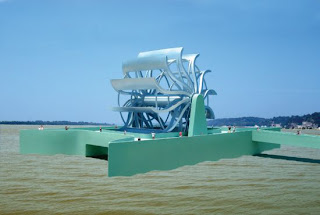
ABSTRACT
Electricity generation using FHT works
on the basic principle of Hydroelectric Generator. Water power is the
combination of HEAD and FLOW. Head and
Flow are the two most important things you need to know about the site. Every
aspect of a hydro system revolves around Head and Flow. The generation of
electricity is simply the conversion of one form of power to another. The
turbine converts water power into rotational power at its shaft, which is then
converted to electrical power by the generator. Hydro-power converts the energy
in flowing water into electricity. The volume of water flow determines the
quantity of electricity generated and the amount of "head" (the
height from turbines in the power plant to the water surface) created by the
dam. The greater the flow and head, the more electricity produced. A typical hydro-power plant includes a dam, reservoir, penstocks (pipes), a powerhouse and
an electrical power substation. The dam stores water and creates the head;
penstocks carry water from the reservoir to turbines inside the powerhouse; the
water rotates the turbines, which drive generators that produce electricity.
The electricity is then transmitted to a substation where transformers increase
voltage to allow transmission to homes, businesses and factories.
Introduction
Our group has selected the Hydroelectric Generator using floating turbine concept in order to utilize knowledge and experience obtained from different power engineering classes. The most difficult part of the project will be synchronizing the speed at which we can spin the turbine with the optimal speed of the motor/generator. We may need to boost the signal from the pump in order to obtain our goals. This project will be exciting because it will allow us to design and build a small hydro-generation system. This system will give insight into the complex task of finding a reliable energy source for future use.
How Hydropower Works? Hydropower converts the energy in flowing water into electricity. The volume of water flow determines the quantity of electricity generated and the amount of "head" (the height from turbines in the power plant to the water surface) created by the dam. The greater the flow and head, the more electricity produced.
A typical hydropower plant includes a dam, reservoir,
penstocks (pipes), a powerhouse and an electrical power substation. The dam
stores water and creates the head; penstocks carry water from the reservoir to
turbines inside the powerhouse; the water rotates the turbines, which drive
generators that produce electricity. The electricity is then transmitted to a
substation where transformers increase voltage to allow transmission to homes,
businesses and factories.
Types of Hydropower Plants
Conventional: Most hydropower plants are conventional in design, meaning they use one-way water flow to generate electricity. There are two categories of conventional plants, run-of-river and storage plants.
Run-of-river plants - These plants use little, if any, stored water to provide water flow through the turbines. Although some plants store a day or week's worth of water, weather changes, especially seasonal changes, cause run-of-river plants to experience significant fluctuations in power output.
Storage plants - These plants have enough storage capacity to off-set seasonal fluctuations in water flow and provide a constant supply of electricity throughout the year. Large dams can store several years worth of water.
Pumped Storage: In contrast to conventional hydropower plants, pumped storage plants reuse water. After water initially produces electricity, it flows from the turbines into a lower reservoir located below the dam. During off-peak hours (periods of low energy demand), some of the water is pumped into an upper reservoir and reused during periods of peak-demand.
Building Hydropower Plants Most hydropower plants are built through federal or local agencies as part of a multi-purpose project. In addition to generating electricity, dams and reservoirs provide flood control, water supply, irrigation, transportation, recreation and refuges for fish and birds. Private utilities also build hydropower plants, although not as many as government agencies.
Overview
System
Components:
An intake collects the water and
a pipeline delivers it to the turbine, The turbine converts the water's energy
into mechanical shaft power. The turbine drives the generator which converts
shaft power into electricity. In an AC system, this power goes directly to the
loads. In a battery-based system, the power is stored in batteries, which feed
the loads as needed. Controllers may be required to regulate the system.
Pipeline: Most hydro systems require a pipeline to feed water to the turbine. The exception is a propeller machine with an open intake. The water should pass first through a simple filter to block debris that may clog or damage the machine. The intake should be placed off to the side of the main water flow to protect it from the direct force of the water and debris during high flows. It is important to use a pipeline of sufficiently large diameter to minimize friction losses from the moving water. When possible, the pipeline should be buried. This stabilizes the pipe and prevents critters from chewing it. Pipelines are usually made from PVC or polyethylene although metal or concrete pipes can also be used.
Turbines:
Although
traditional waterwheels of various types have been used for centuries, they
aren't usually suitable for generating electricity: They are heavy, large and
turn at low speeds. They require complex gearing to reach speeds to run an
electric generator. They also have icing problems in cold climates. Water
turbines rotate at higher speeds, are lighter and more compact. Turbines are
more appropriate for electricity generation and are usually more efficient.
There are
two basic kinds of turbines: Impulse and Reaction.
Impulse
machines use a nozzle at the end of the pipeline that converts the water under
pressure into a fast moving jet. This jet is then directed at the turbine wheel
(also called the runner), which is designed to convert as much of the jet's
kinetic energy as possible into shaft power. Common impulse turbines are
Pelton, Turgo and Cross-Flow.
In
reaction turbines, the energy of the water is converted from pressure to
velocity within the guide vanes and the turbine wheel itself. Some lawn
sprinklers are reaction turbines. They spin themselves around as a reaction to
the action of the water squirting from the nozzles in the arms of the rotor.
Examples of reaction turbines are propeller and Francis turbines.
Turbine Applications In the family of impulse machines, the Pelton is used for the lowest flows and highest heads. The cross-flow is used where flows are highest and heads are lowest. The Turgo is used for intermediate conditions. Propeller (reaction) turbines can operate on as little as two feet of head. A Turgo requires at least four feet and a Pelton needs at least ten feet. These are only rough guidelines with overlap in applications.
The
Cross-Flow (impulse) turbine is the only machine that readily lends itself to
user construction. They can be made in modular widths and variable nozzles can
be used.
Most
developed sites now use impulse turbines. These turbines are very simple and
relatively cheap. As the stream flow varies, water flow to the turbine can be
easily controlled by changing nozzle sizes or by using adjustable nozzles. In
contrast, most small reaction turbines cannot be adjusted to accommodate
variable water flow. Those that are adjustable are very expensive because of
the movable guide vanes and blades they require. If sufficient water is not
available for lull operation of a reaction machine, performance suffers
greatly.
An
advantage of reaction machines is that they can use the full head available at
a site. An impulse turbine must be mounted above the tailwater level and the
effective head is measured down to the nozzle level. For the reaction turbine,
the full available head is measured between the two water levels while the
turbine can be mounted well above the level of the exiting water. This is
possible because the "draft-tube" used with the machine recovers some
of the pressure head after the water exits the turbine. This cone-shaped tube
converts the velocity of the flowing water into pressure as it is decelerated
by the draft tube's increasing cross section. This creates suction on the
underside of the runner.
Centrifugal
pumps are sometimes used as practical substitutes for reaction turbines with
good results. They can have high efficiency and are readily available (both new
and used) at prices much lower than actual reaction turbines. However, it may
be difficult to select the correct pump because data on its performance as a
turbine are usually not available or are not straightforward. One reason more
reaction turbines are not in use is the lack of available machines in small
sizes. There are many potential sites with 2 to, 10 feet of head and high flow
that are not served by the market.
Power capacity
Calculation
of Energy and Power
Force = mass x acceleration F = ma (Typical Unit -Newton’s)
Energy = Work (W) = Force (F) x Distance (d) (Typical unit
– Joules)
Power = P = W / time (t) (Typical unit –Watts)
Power = Torque
(Q) x Rotational Speed (Ω)
Kinetic
Energy in the Water
Kinetic Energy = Work = ½MV2
Where:
M= mass of moving object
V = velocity of moving object
Mass
of moving water
M = density (ρ) x volume (Area x distance)
= ρ x A x d
= (kg/m3) (m2) (m)
= kg
= 1000 x 0.196x 0.5
=
98 kg
Water
Velocity v = 2 m/s
P = 0.5 x
A x ρ x v3
Advantages
and Disadvantages
Advantages of the Hydroelectric
Generator using FHP:
·
Non-polluting generation for operation in enclosed areas.
·
Readily available supply of fuel (water).
·
Provides an alternative to gasoline-powered generators.
·
Save money by using water instead of gasoline.
·
No flammable components.
·
Emergency backup power supply.
Disadvantages of the Hydroelectric
Generator using FHP:
·
Not a completely reliable source of
electricity.
·
Completely depends upon water supply.
·
As water is used, there is danger of
corrosion in the long run.
Benefits: Hydropower is a clean, domestic and renewable source of energy. Hydropower plants provide inexpensive electricity and produce no pollution. And, unlike other energy sources such as fossil fuels, water is not destroyed during the production of electricity—it can be reused for other purposes.
Obstacles: Hydropower plants can significantly impact the surrounding area—reservoirs can cover towns, scenic locations and farmland, as well as affect fish and wildlife habitat. To mitigate impact on migration patterns and wildlife habitats, dams maintain a steady stream flow and can be designed or retrofitted with fish ladders and fish ways to help fish migrate upstream to spawn.
What is COANDA EFFECT?
The design of the FLOATING HYDRO TURBINE
evolved from this amphibious vehicle. Built in the 70's.
On water at speeds of less than 4 mph (6.5
kph) everything rolled along just fine but when the throttle was opened past
this point instead of going faster the vehicle started going down.
This is the Coanda Effect.
How it works
Clearly some method of overcoming this
down-force was needed. A method of breaking the water suction.
A 3 foot (91cm) diameter ball with
axle bearings was made. When towed behind a boat it was found that at
speeds over 4 mph (6.5 kph) the ball began to dive and would behave more
like an anchor even though it was mounted on free rotating axle bearings and was
otherwise very bouyant.
After adding a chevron tread pattern
similar to that now shown on the hydro-electric-barrel a speed of 16 mph (26
kph) was acheived and the ball span readily on the water surface.
A straight tread did not solve the problem
effectively due to downward force applied by the treads as they emerged from
the water.
About FHT
The FHT could be used to produce both wave energy and energy from
tidal current at the same time.
The barrel rolls on the waves, rising and falling to drive both hub
and linear or flywheel permanent magnet generators.
BENEFITS
Small operation
Easy to transport and install.
Friendly with environment due to shallow draft.
Cost is less compared to water & solar.
DISADVANTAGES
Adjusts to water level.
Need more power? Install another FHT.
Does not interrupt river flow and can roll over waves.
Many more applications
Remote area power supply for battery charging, lighting, irrigation,
refrigeration, monitoring.
Potential for grants and feed-in tariffs.
No comments:
Post a Comment
leave your opinion