
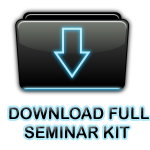
connects the two shafts so that they can either be locked together and spin at the same speed (engaged), or be decoupled and spin at different speeds (disengaged).
TYPES OF CLUTCH
DOG CLUTCH
A dog clutch is a type of clutch that couples two rotating shafts or other rotating components not by friction but by interference. The two parts of the clutch are designed such that one will push the other, causing both to rotate at the same speed and will never slip.
Dog clutches are used where slip is undesirable and/or the clutch is not used to control torque. Without slippage, dog clutches are not affected by wear in the same way that friction clutches are.
Dog clutches are used inside manual automotive transmissions to lock different gears to the rotating input and output shafts. A synchromesh arrangement ensures smooth engagement by matching the shaft speeds before the dog clutch is allowed to engage.
Wet and dry
A 'wet clutch' is immersed in a cooling lubricating fluid, which also keeps the surfaces clean and gives smoother performance and longer life. Wet clutches; however, tend to lose some energy to the liquid. A 'dry clutch', as the name implies, is not bathed in fluid. Since the surfaces of a wet clutch can be slippery (as with a motorcycle clutch bathed in engine oil), stacking multiple clutch disks can compensate for the lower coefficient of friction and so eliminate slippage under power when fully engaged.
Cone clutch
A cone clutch serves the same purpose as a disk or plate clutch. However, instead of mating two spinning disks, the cone clutch uses two conical surfaces to transmit torque by friction.
The cone clutch transfers a higher torque than plate or disk clutches of the same size due to the wedging action and increased surface area.
Cone clutches are generally now only used in low peripheral speed applications although they were once common in automobiles and other combustion engine transmissions.
They are usually now confined to very specialist transmissions in racing, rallying, or in extreme off-road vehicles, although they are common in power boats. This is because the clutch doesn't have to be pushed in all the way and the gears will be changed quicker.
Centrifugal clutch
A centrifugal clutch is a clutch that uses centrifugal force to connect two concentric shafts, with the driving shaft nested inside the driven shaft.
Centrifugal clutches are often used in mopeds, lawnmowers, go-karts, chainsaws, and mini bikes.
Fluid coupling
A fluid coupling is a hydrodynamic device used to transmit rotating mechanical power. It has been used in automobile transmissions as an alternative to a mechanical clutch. It also has widespread application in marine and industrial machine drives, where variable speed operation and/or controlled start-up without shock loading of the power transmission system is essential.
Torque limiter
It is also known as safety clutch. A torque limiter is an automatic device that protects mechanical equipment, or its work, from damage by mechanical overload. A torque limiter may limit the torque by slipping (as in a friction plate slip-clutch), or uncouple the load entirely (as in a shear pin). The action of a torque limiter is especially useful to limit any damage due to crash stops and jams.
Torque limiters may be packaged as a shaft coupling or as a hub for sprocket or sheave. A torque limiting device is also known as an overload clutch.
Electromagnetism
What is an electromagnet?
An electromagnet can be made by sending current through a coil of wire wound around an iron core.
When current passes through a conductor, magnetic field will be generated around the conductor and the conductor become a magnet. This phenomenon is called electromagnetism. Since the magnet is produced electric current, it is called the electromagnet.
In short, when current flow through a conductor, magnetic field will be generated. When the current ceases, the magnetic field disappear.
The direction of the magnetic field formed by a current carrying straight wire can be determined by the Right Hand Grip Rule or the Maxwell Screw Rule.
What is magnetic field pattern?
A magnetic field pattern can be represented by field lines that show the shape of the field.
Magnetic field lines which are closed together represents strong field.
Field direction is defined as the direction indicated by a compass needle placed in the magnetic field.
Force on current carrying conductor
If a current carrying conductor is placed in a magnetic field produced by permanent magnets, then the field due to current carrying conductor and the permanent magnets interact and cause the a force to be exerted on the conductor . The force on the current carrying conductor in a magnetic field depends on:-
1. The flux density of the field, B teslas
2. The strength of current, I amp
3. The length of the conductor perpendicular to the magnetic field
4. The direction of the field and current
When the magnetic field, the current and the conductor are mutually perpendicular to each other then force exerted:
F=IBL Newton
When the conductor and magnetic field are at an angle, then the force exerted:
F=IBLsinα
Where;
I=current, B magnetic field, L=Length of conductor
α-angle between conductor and magnetic field.
Right Hand Grip Rule
Grip the wire with the right hand, with the thumb pointing along the direction of the current. The other fingers give the direction of the magnetic field around the wire.
The Maxwell's Screw Rules
The Maxwell Screw Rules sometime is also called the Maxwell's Corkscrew Rule. Imagine a right handed screw being turn so that it bores its way in the direction of the current in the wire. The direction of rotation gives the direction of the magnetic field.
Electromagnetic clutch
Electromagnetic clutches operate electrically, but transmit torque mechanically. This is why they used to be referred to as electro-mechanical clutches.
CONSTRUCTION
A horseshoe magnet has a north and south pole. If a piece of carbon steel contacts both poles, a magnetic circuit is created. In an electromagnetic clutch, the north and south pole is created by a coil shell and a wound coil. In a clutch, when power is applied, a magnetic field is created in the coil. This field (flux) overcomes an air gap between the clutch rotor and the armature. This magnetic attraction, pulls the armature in contact with the rotor face. The frictional contact, which is being controlled by the strength of the magnetic field, is what causes the rotational motion to start. The torque comes from the magnetic attraction, of the coil and the friction between the steel of the armature and the steel of the clutch rotor. For many industrial clutches, friction material is used between the poles. The material is mainly used to help decrease the wear rate, but different types of material can also be used to change the coefficient of friction (torque for special applications). For example, if the clutch is required to have an extended time to speed or slip time, a low coefficient friction material can be used and if a clutch is required to have a slightly higher torque (mostly for low rpm applications), a high coefficient friction material can be used.
In a clutch, the electromagnetic lines of flux have to pass into the rotor, and in turn, attract and pull the armature in contact with it to complete clutch engagement. Most industrial clutches use what is called a single flux, two pole design. Mobile clutches of other specialty electromagnetic clutches can use a double or triple flux rotor. The double or trip flux refers to the number of north/south flux paths, in the rotor and armature.
This means that, if the armature is designed properly and has similar banana slots, what occurs is a leaping of the flux path, which goes north south, north south. By having more points of contact, the torque can be greatly increased. In theory, if there were 2 sets of poles at the same diameter, the torque would double in a clutch. Obviously, that is not possible to do, so the points of contact have to be at a smaller inner diameter. Also, there are magnetic flux losses because of the bridges between the banana slots. But by using a double flux design, a 30%-50% increase in torque, can be achieved, and by using a triple flux design, a 40%-90% in torque can be achieved. This is important in applications where size and weight are critical, such as automotive requirements.
The coil shell is made with carbon steel that has a combination of good strength and good magnetic properties. Copper (sometimes aluminium) magnet wire, is used to create the coil, which is held in shell either by a bobbin or by some type of epoxy/adhesive.
To help increase life in applications, friction material is used between the poles on the face of the rotor. This friction material is flush with the steel on the rotor, since if the friction material was not flush, good magnetic traction could not occur between the faces. Some people look at electromagnetic clutches and mistakenly assume that, since the friction material is flush with the steel that the clutch has already worn down but this is not the case. Clutches used in most mobile applications, (automotive, agriculture, construction equipment) do not use friction material. Their cycle requirements tend to be lower than industrial clutches, and their cost is more sensitive. Also, many mobile clutches are exposed to outside elements, so by not having friction material, it eliminates the possibility of swelling (reduced torque), that can happen when friction material absorbs moisture.
HOW DOES IT WORKS
The clutch has four main parts: field, rotor, armature, and hub (output) . When voltage is applied the stationary magnetic field generates the lines of flux that pass into the rotor. (The rotor is normally connected to the part that is always moving in the machine.) The flux (magnetic attraction) pulls the armature in contact with the rotor (the armature is connected to the component that requires the acceleration), as the armature and the output start to accelerate. Slipping between the rotor face and the armature face continues until the input and output speed is the same (100% lockup). The actual time for this is quite short, between 1/200th of a second and 1 second.
Disengagement is very simple. Once the field starts to degrade, flux falls rapidly and the armature separates. One or more springs hold the armature away from the rotor at a predetermined air gap.
There are actually two engagement times to consider in an electromagnetic clutch. The first one is the time that it takes for a coil to develop a magnetic field, strong enough to pull in an armature. Within this, there are two factors to consider. The first one is the amount of ampere turns in a coil, which will determine the strength of a magnetic field. The second one is air gap, which is the space between the armature and the rotor. Magnetic lines of flux diminish quickly in the air.. Air gap is an important consideration especially with a fixed armature design because as the unit wears over many cycles of engagement the armature and the rotor will create a larger air gap which will change the engagement time of the clutch. In high cycle applications, where registration is important, even the difference of 10 to 15 milliseconds can make a difference, in registration of a machine. Even in a normal cycle application, this is important because a new machine that has accurate timing can eventually see a “drift” in its accuracy as the machine gets older.
The second factor in figuring out response time of a clutch is actually much more important than the magnet wire or the air gap. It involves calculating the amount of inertia that the clutch needs to accelerate. This is referred to as “time to speed”. In reality, this is what the end-user is most concerned with. Once it is known how much inertia is present for the clutch to start then the torque can be calculated and the appropriate size of clutch can be chosen.
Most CAD systems can automatically calculate component inertia, but the key to sizing a clutch is calculating how much inertial is reflected back to the clutch or brake. To do this, engineers use the formula: T = (wk2 × ΔN) / (308 × t) Where T = required torque in lb-ft, WK2 = total inertia in lb-ft2, ΔN = change in the rotational speed in rpm, and t = time during which the acceleration or deceleration must take place.
There are also online sites that can help confirm how much torque is required to accelerate a given amount of inertia over a specific time.
OBJECTIVE
A control circuit is to be designed to control the motor and drive unit. The Design specifications are to be fully implemented. An incomplete circuit and equipments are given and once it is understood appropriate values for the different components should be decided. These values should allow the circuit to perform as specified.
Design Specification
A circuit is to be designed which is
1. Allows the angular speed of the motor to build up to a value of 20 revolutions per minute (rpm), in a time of 2 seconds (s).
2. Maintains the angular speed of 20 rpm for a time of 3s.
3. When clutch pedal is pressed it brings the machine to a halt in a time of 1s.
4. Builds up the angular speed again to 20 rpm, in a time of 2s,
except this time in the opposite direction.
5. Maintains the angular speed of 20 rpm for a time of 6s.
6. Brings the machine to a halt in a time of 1s.
7. Repeats the cycle above (1-6) indefinitely when powered on.
The motor must not be in dynamic braking mode at the same time as it is being driven.
good
ReplyDeletegood
ReplyDeletenice
ReplyDelete