Non-destructive testing (NDT) is a wide group of analysis techniques used in science and industry to evaluate the properties of a material, component or system without causing damage. The terms Nondestructive examination (NDE), Nondestructive inspection (NDI), and Nondestructive evaluation (NDE) are also commonly used to describe this technology. Because NDT does not permanently alter the article being inspected, it is a highly-valuable technique that can save both money and time in product evaluation, troubleshooting, and research.
Non-destructive Testing is one part of the function of Quality Control and is Complementary to other long established methods. By definition non-destructive testing is the testing of materials, for surface or internal flaws or metallurgical condition, without interfering in any way with the integrity of the material or its suitability for service.
The technique can be applied on a sampling basis for individual investigation or may be used for 100% checking of material in a production quality control system. Whilst being a high technology concept, evolution of the equipment has made it robust enough for application in any industrial environment at any stage of manufacture - from steel making to site inspection of components already in service. A certain degree of skill is required to apply the techniques properly in order to obtain the maximum amount of information concerning the product, with consequent feed back to the production facility. Non-destructive Testing is not just a method for rejecting substandard material; it is also an assurance that the supposedly good is good. The technique uses a variety of principles; there is no single method around which a black box may be built to satisfy all requirements in all circumstances.
What follows is a brief description of the methods most commonly used in industry, together with details of typical applications, functions and advantages.


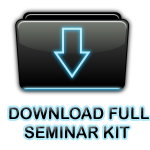
The methods Covered are:
• Radiography
• Magnetic Particle Crack Detection
• Dye Penetrate Testing
• Ultrasonic Flaw Detection
• Eddy Current and Electro-magnetic Testing
However, these are by no means the total of the principles available to the N.D.T. Engineer. Electrical potential drop, Sonics, infra-red, acoustic emission and Spectrograph, to name but a few, have been used to provide information that the above techniques have been unable to yield, and development across the board Continues.
Definitions
The following definitions apply
Testing: Testing or examination of a material or component in accordance with this Classification Note, or a standard, or a specification or a procedure in order to detect, locate, measure and evaluate flaws.
Defect: One or more flaws whose aggregate size, shape, orientation, location or properties do not meet specified requirements and are rejectable.
Discontinuity: A lack of continuity or cohesion; an intentional or unintentional interruption in the physical structure or configuration of a material or component
Flaw: An imperfection or discontinuity that may be detectable by non-destructive testing and is not necessarily reject able.
Indication: Evidence of a discontinuity that requires interpretation to determine its significance
False indication: An indication that is interpreted to be caused by a discontinuity at a location where no discontinuity exists.
Non relevant indication: An indication that is caused by a condition or type of discontinuity that is not reject able. False indications are non-relevant
Imperfections: A departure of a quality characteristic from its intended condition.
Internal imperfections: Imperfections that are not open to a surface or not directly accessible.
Quality level: Fixed limits of imperfections corresponding to the expected quality in a specific object. The Limits are determined with regard to type of imperfection, their amount and their actual dimensions.
Acceptance level: Prescribed limits below which a component is accepted.
Planar discontinuity: Discontinuity having two measurable dimensions
Non-planar discontinuity: Discontinuity having three measurable dimensions.
Abbreviations
· ET Eddy current testing
· MT Magnetic particle testing
· PT Penetrant testing
· RT Radiographic testing
· UT Ultrasonic testing
· VT Visual testing
· HAZ Heat affected zone
· WPS Welding Procedure Specification
· TMCP Thermo mechanically controlled processed
· NDT Non-destructive testing.
Where to apply NDE Methods:
There are NDE application at almost any stage in the production or life cycle of a component.
There are NDE application at almost any stage in the production or life cycle of a component.
- To assist in product development
- To screen or sort incoming materials
- To monitor, improve or control manufacturing processes
- To verify proper processing such as heat treating
- To verify proper assembly
- To inspect for in-service damage
Uses of NDE Methods
· Flaw Detection and Evaluation
· Leak Detection
· Location Determination
· Dimensional Measurements
· Structure and Microstructure Characterization
· Estimation of Mechanical and Physical Properties
· Stress (Strain) and Dynamic Response Measurements
· Material Sorting and Chemical Composition Determination
HISTORY OF NDT-INSTRUMENTATION
After World War II the emerging modern industry needed more and more testing equipment for the production of flawless components. Therefore, instruments for NDT were developed, produced in quantities and continuously improved. The first NDT-method coming into industrial application was the X-Ray Technique.
X-Ray Technique
Already 1895 Wilhelm Conrad Röntgen discovered "An Unknown Kind of Radiation" which were named in all German speaking countries after him. In his first publication he described all effects including possible flaw detection. At that time industry did not yet need this invention but medicine did. So medical equipment was developed, used and produced in quantities. The only effect Röntgen could not foresee was that X-rays harm human health. Before radiation protection became introduced, many persons lost their life. Early technical X-ray applications in Germany were realized by Richard Seifert around 1930. He improved medical equipment, cooperated with welding-institutes and built up the small company founded by his father to a world-wide respected name: Richard Seifert Hamburg 13. He got competition by Siemens and C.H.F. Muller, part of the Philips-organization, who already worked in the medical field. Seifert died in 1969, but his company kept leadership in technical X-ray-application under the direction of his youngest daughter Elisabeth Samish. Radiation testing can also be carried out with radioactive isotopes. This was discovered by Mme. Curie. She, born as Maria Sklodowska in Warscaw received the Nobel-prize for physics in 1903 together with her husband Pierre Curie and Henri Becquerel. This was the second award after Rontgen’s in 1901. Also radioactive isotopes were initially used for medical applications. In Germany Rudolf Berthold and Otto Vaupel applied them after 1933 to welded joints. After World War II Arturo Gilardoni in Italy, Drenk and Andreasen in Denmark developed X-ray-equipment, Kurt Sauerwein portable isotope-containers in Germany.
Magnetic particle crack detection
Magnetic particle crack detection was executed even earlier than X-ray testing. The Englishman S.M. Saxby already in 1868 and the American William Hoke in 1917 tried to find cracks in gun barrels by magnetic indications. Real industrial application was made by Victor de Forest and Foster Doane after 1929. They formed 1934 a company with the name Magnaflux in 1934, famous world-wide until today. The first European who built a magnetic particle crack detector was an Italian in 1932: Giraudi. His machine was named "Metalloscopio". In Germany Berthold and Vaupel applied MP-technique to welded constructions. Their equipment was produced by Ernst Heubach. Bruno Suschyzki sold this equipment. He invented swinging field MP-testing.In Berlin too E.A.W. Müller designed MP-testing machines for Siemens. In Prague the Seifert-representative Karasek began with similar production.After World War II Wilhelm Tiede, a former Seifert-employee, started his own company in Southern Germany.
Through the Seifert-organisation he had connections to Karasek who emigrated to Brazil in 1948 after the communistic revolution in Cechoslovakia. There he continued production of MP-machines.Starting with dry-powder methods two more companies entered this market in the late Fifties: Karl Deutsch in Germany and CGM (Carlo Gianni Milano) in Italy.
Penetrate Testing
Penetrant Testing started also in second half of 19th century. The first people who applied the "Oil and Whiting"-process for crack detection to railway-components are unknown. The method was replaced by the upcoming MP-technique. Just before and during World War II the fast growing aircraft-industry used more and more nonmagnetic light metals, which could not be tested with MP. So independent of each other Magnaflux together with the brothers Switzer in USA, Brent Chemicals in GB, Adler (Blohm & Voss) and Klumpf (Junkers) in Germany started production of fluorescent and dye penetrants.
Eddy Current Testing
Eddy Current Testing has old roots as well. The French Dominique Arago discovered the phenomenon during the first half of 19th century. The principle was explained by and named after Leon Foucault. Many proposals for application were made until Friedrich Förster brought this method to industrial use. He worked since 1933 for the Kaiser-Wilhelm-Institute and there he developed instruments for measuring conductivity and to sort out mixed-up ferrous components. In 1948 he founded his own company in Reutlingen which grew up with eddy current testing (ET). Institut Dr. Förster became market-leader worldwide for many decades. In Sweden Anders Arnelo started similar developments at Svenska Metalverken (SM). He solved the problem to test hot wires and invented the pre-magnetization for ET of ferritic bars.Other companies followed later: Magnaflux, Hentschel, Law and Zetec in the USA, Rohmann and Prüftechnik Busch & Partner in Germany, Bergstrand in Sweden and Hocking in Britain.
Ultrasonic Testing
Came latest into industrial use. The methods of exciting ultrasound were discovered already in 1847 by James Precott Joule and in 1880 by Pierre Curie and his brother Paul Jacques. Not earlier than 1912 a first application was proposed after the "Titanic" had sunk. The Englishman Richardson claimed the identification of icebergs by ultrasound in his patent applications. In France Chilowski and Langevin started their development to detect submarines by ultrasound during World War I. In 1929 the Russian Sokolov proposed to use ultrasound for testing castings. In Berlin Pohlman realized in 1937 an image-cell to indicate the differences of ultrasound-energy similar to a X-ray image-screen.
The detection of laminations in plates and fine non-metallic inclusions in hot-rolled profiles became necessary during World War II. The already existing NDT-methods - X-rays, MP, PT and ET - were unable to solve these problems.Industrial uses of ultrasonic testing started simultaniously in three countries: USA, GB and Germany. The key-persons, Floyd Firestone, Donald O. Sproule and Adolf Trost had no knowledge of each other as they worked strictly in secret. Not even their patent-applications were published. Sproule and Trost used transmission-technique with seperate transmitter- and receiver-probes. Trost invented the so-called "Trost-Tonge". The 2 probes were contacted on opposite sides of a plate, held in same axis by a mechanical device - the tonge - and coupled to both surfaces by continuously flowing water. Sproule placed the 2 probes on the same side of the workpiece. So he invented double-crystal probes. But it has to be mentioned that he used this combination also with variing distance from each other. Firestone was the first to realize the reflection-technique. He modified a radar instrument and developed a transmitter with short pulses and an amplifier with short dead-zone.Sproule and Firestone found industrial partners for their instruments: Kelvin-Hughes and Sperry Inc.In Germany 1949 two persons received information about the Firestone-Sperry-Reflectoscope by publications in technical papers: Josef Krautkrämer in Cologne and Karl Deutsch in Wuppertal. Both started developments - without knowledge of each other. Josef Krautkrämer and his brother Herbert were physicists, working in the field of oscilloscopes. They could develop ultrasonic instruments alone. Karl Deutsch, a mechanical engineer needed a partner for the electronics and found him with Hans-Werner Branscheid who had got some technical experience in radar-technique during the war. Within only one year both young and tiny companies could present their UT-flaw-detectors, starting a competition still existing today.Later on more UT-units came on the international markets: Siemens and Lehfeldt in Germany, Kretztechnik in Austria, Ultrasonique in France and Ultrasonoscope in Britain. They all stopped their production before the 70-ies, Kelvin-Hughes also stopped at the same time, Sperry was later renamed Automation Ind., around 1995.
Krautkrämer became world-wide market-leader in the early 60-ies and has kept this position until today. Besides Karl Deutsch new names came up: Nukem in Germany, Panametrics and Stavely (after Sonic and Harisonic) in USA, Sonatest and Sonomatic in GB, Gilardoni in Italy and Mitsubishi in Japan.Today more than 50 companies are active in industrial NDT. They are still working under strong competition to the benefit of their customers on their way to improve the quality of industrially produced parts.
A BRIEF DESCRIPTION OF NDT TECHNIQUES
Radiography
This technique is suitable for the detection of internal defects in ferrous and nonferrous metals and other materials. X-rays, generated electrically, and Gamma rays emitted from radio-active isotopes, are penetrating radiation which is differentially absorbed by the material through which it passes; the greater the thickness, the greater the absorption. Furthermore, the denser the material the greater the absorption. X and Gamma rays also have the property, like light, of partially converting silver halide crystals in a photographic film to metallic silver, in proportion to the intensity of the radiation reaching the film, and therefore forming a latent image. This can be developed and fixed in a similar way to normal photographic film. Material with internal voids is tested by placing the subject between the source of radiation and the film. The voids show as darkened areas, where more radiation has reached the film, on a clear background. The principles are the same for both X and Gamma radiography.
In X-radiography the penetrating power is determined by the number of volts applied to the X-Ray tube - in steel approximately 1000 volts per inch thickness is necessary. In Gamma radiography the isotope governs the penetrating power and is unalterable in each isotope. Thus Iridium 192 is used for 1/2" to 1" steel and Caesium 134 is used for 3/4" to 21/2" steel. In X-radiography the intensity, and therefore the exposure time, is governed by the amperage of the cathode in the tube. Exposure time is usually expressed in terms of milliampere minutes. With Gamma rays the intensity of the radiation is set at the time of supply of the isotope. The intensity of radiation from isotopes is measured in Becquerel’s and reduces over a period of time. The time taken to decay to half the amount of curies is the half life and is characteristic of each isotope. For example, the half life of Iridium 192 is 74 days, and Caesium 134 is 2.1 years. The exposure factor is a product of the number of curies and time, usually expressed in curie hours. The time of exposure must be increased as the isotope decays - when the exposure period becomes uneconomical the isotope must be renewed. As the isotope is continuously emitting radiation it must be housed in a container of depleted uranium or similar dense shielding material, whilst not exposed to protect the environment and personnel.
To produce an X or Gamma radiograph, the film package (comprising film and intensifying screens - the latter being required to reduce the exposure time – enclosed in a light tight cassette) is placed close to the surface of the subject. The source of radiation is positioned on the other side of the subject some distance away, so that the radiation passes through the subject and on to the film. After the exposure period the film is removed, processed, dried, and then viewed by transmitted light on a special viewer. Various radiographic and photographic accessories are necessary, including such items as radiation monitors, film markers, image quality indicators, darkroom equipment, etc. Where the last is concerned there are many degrees of sophistication, including fully automatic processing units. These accessories are the same for both X and Gamma radiography systems. Also required are such consumable items as radiographic film and processing chemicals.
The part is placed between the radiation source and a piece of film. The part will stop some of the radiation. Thicker and denser area will stop more of the radiation
Advantages of Radiography
1. Information is presented pictorially.
2. A permanent record is provided which may be viewed at a time and place
3. Distant from the test.
4. Useful for thin sections.
5. Sensitivity declared on each film.
6. Suitable for any material.
Disadvantages of Radiography
1. Generally an inability to cope with thick sections.
2. Possible health hazard.
3. Need to direct the beam accurately for two-dimensional defects.
4. Film processing and viewing facilities are necessary, as is an exposure compound.
5. Not suitable for automation, unless the system incorporates fluoroscopy with
6. an image intensifier or other electronic aids
7. Not suitable for surface defects.
8. No indication of depth of a defect below the surface
Magnetic Particle Inspection
This method is suitable for the detection of surface and near surface discontinuities in magnetic material, mainly ferrite steel and iron. An Illustration of the Principle of Magnetic Particle Inspection
The principle is to generate magnetic flux in the article to be examined, with the flux lines running along the surface at right angles to the suspected defect. Where the flux lines approach a discontinuity they will stay out in to the air at the mouth of the crack. The crack edge becomes magnetic attractive poles North and South. These have the power to attract finely divided particles of magnetic material such as iron fillings. Usually these particles are of an oxide of iron in the size range 20 to 30 microns, and are suspended in a liquid which provides mobility for the particles on the surface of the test piece, assisting their migration to the crack edges. However, in some instances they can be applied in a dry powder form. The particles can be red or black oxide, or they can be coated with a substance, which fluoresces brilliantly under ultra-violet illumination (black light). The object is to present as great a contrast as possible between the crack indication and the material background. The technique not only detects those defects which are not normally visible to the unaided eye, but also renders easily visible those defects which would otherwise require close scrutiny of the surface. There are many methods of generating magnetic flux in the test piece, the simplest one being the application of a permanent magnet to the surface, but this method cannot be controlled accurately because of indifferent surface contact and deterioration in magnetic strength. Modern equipments generate the magnetic field electrically either directly or indirectly.
In the direct method a high amperage current is passed through the subject and magnetic flux is generated at right angles to the current flow. Therefore the current flow should be in the same line as the suspected defect. If it is not possible to carry out this method because of the orientation of the defect, then the indirect method must be used.
This can be one of two forms:
1. Passing a high current through a coil that encircles the subject.
2. Making the test piece form part of a yoke, this is wound with a current carrying coil. The effect is to pass magnetic flux along the part to reveal transverse and circumferential defects.
If a bar with a length much greater than its diameter is considered, then longitudinal defects would be detected by current flow and transverse and circumferential defects by the indirect method of an encircling coil or magnetic flux flow. Subjects in which cracks radiating from a hole are suspected can be tested by means of the threading bar technique, whereby a current carrying conductor is passed through the hole and the field induced is cut by any defects. Detection of longitudinal defects in hollow shafts is a typical application of the threader bar technique. The electricity used to generate the magnetic flux in any of these methods can be alternating current, half wave rectified direct current or full wave rectified direct current. A.C. generated magnetic flux, because of the skin effect, preferentially follows the contours of the surface and does not penetrate deeply into the material. H.W.D.C. penetrates more deeply but is inclined not to follow sharp changes in section. H.W.D.C. is useful for the detection of slightly subsurface defects. The pulsing effect of A.C. and H.W.D.C. gives additional mobility to the indicating particles. D.C. penetrates even more deeply but does not have this facility. Furthermore, demagnetizing of the material after D.C. magnetizing is far more difficult than after A.C. magnetizing. Normally, to ensure that a test piece has no cracks, it is necessary to magnetize it in at least two directions and after each magnetizing - and ink application – visually examine the piece for crack indications. Since this double process, which would include adjustment of the magnetizing equipment controls in between each magnetizing takes time it is obviously advantageous to have the facility to reduce the time required.
The recent development of the Swinging Field method of multi-directional magnetizing will indicate all defects, regardless of their orientation on the surface, with one magnetizing shot and therefore requires only one inspection. (Please refer to our paper entitled Faster Magnetic Crack Detection using the Multi-directional Swinging Field Method).
Basically magnetic crack detection equipment takes two forms. Firstly, for test pieces which are part of a large structure, or pipes, heavy castings, etc. which cannot be moved easily, the equipment takes the form of just a power pack to generate a high current. This current is applied to the subject either by contact prods on flexible cables or by an encircling coil of cable. These power packs can have variable amperages up to a maximum of 2000 Amps for portable units, and up to 10,000 Amps for mobile equipments. Both A.C. and H.W.D.C. magnetising current is available. The indicating material is applied by means of a spray and generally the surplus runs to waste. For factory applications on smaller more manageable test pieces the bench type of equipment, as represented by our EUROMAG range, is normally preferred. This consists of a power pack similar to those described above, an indicating ink system which recirculates the fluid, and facilities to grip the work piece and apply the current flow or magnetic flux flow in a more methodical, controlled manner. The work pieces are brought to the equipment and can be individually tested. Subjects up to approximately 100" long can be accommodated is such equipments and can be loaded by crane if necessary. This type of universal equipment is ideally suited to either investigative work or routine quality control testing. These bench type equipments often incorporate a canopy to prevent direct light falling on the subject so that ultra-violet fluorescent material can be used to the best effect. The indicating particles may be suspended in very thin oil (kerosene) or water. In some circumstances the indicating medium can be applied dry. These equipments are suited to production work and in certain circumstances can be automated to the extent of loading, magnetising, inking and unloading. The work pieces still have to be viewed by eye for defect indications. Specialised equipments are also frequently manufactured to test a particular size and type of test piece.
Advantages of Magnetic Particle Crack Detection
1. Simplicity of operation and application.
2. Quantitative.
3. Can be automated, apart from viewing. (Though modern developments in automatic defect recognition can be used in parts of simple geometry e.g. billets and bars. In this case a special camera captures the defect indication image and processes it for further display and action)
Disadvantages of Magnetic Particle Crack Detection
1. Restricted to ferromagnetic materials.
2. Restricted to surface or near surface flaws.
3. Not fail safe in that lack of indication could mean no defects or process not carried out properly.
Dye Penetrate Testing
This method is frequently used for the detection of surface breaking flaws in non ferromagnetic materials. The subject to be examined is first of all chemically cleaned, usually by vapour phase, to remove all traces of foreign material, grease, dirt, etc. from the surface generally, and also from within the cracks. Next the penetrate (which is a very fine thin oil usually dyed bright red or ultra-violet fluorescent) is applied and allowed to remain in contact with the surface for approximately fifteen minutes. Capillary action draws the penetrate into the crack during this period.
The surplus penetrate on the surface is then removed completely and thin coating of powdered chalk is applied. After a further period (development time) the chalk draws the dye out of the crack, rather like blotting paper, to form a visual, magnified in width, indication in good contrast to the background. The process is purely a mechanical/chemical one and the various substances used may be applied in a large variety of ways, from aerosol spray cans at the most simple end to dipping in large tanks on an automatic basis at the other end. The latter system requires sophisticated tanks, spraying and drying equipment but the principle remains the same.
Advantages of Dye Penetrate Testing
1. Simplicity of operation.
2. Best method for surface breaking cracks in non-ferrous metals.
3. Suitable for automatic testing, with reservation concerning viewing.
(See automatic defect recognition in Magnetic Particle Inspection)
Disadvantages of Dye Penetrate Testing
1. Restricted to surface breaking defects only.
2. Decreased sensitivity.
3. Uses a considerable amount of consumables.
Ultrasonic Flaw Detection
This technique is used for the detection of internal and surface (particularly distant surface) defects in sound conducting materials. The principle is in some respects similar to echo sounding. A short pulse of ultrasound is generated by means of an electric charge applied to a piezoelectric crystal, which vibrates for a very short period at a frequency related to the thickness of the crystal. In flaw detection this frequency is usually in the range of one million to six million times per second (1 MHz to 6 MHz). Vibrations or sound waves at this frequency have the ability to travel a considerable distance in homogeneous elastic material, such as many metals with little attenuation. The velocity at which these waves propagate is related to the Young’s Modulus for the material and is characteristic of that material. For example the velocity in steel is 5900 meters per second, and in water 1400 meters per second. Ultrasonic energy is considerably attenuated in air, and a beam propagated through a solid will, on reaching an interface (e.g. a defect, or intended hole, or the back wall) between that material and air reflect a considerable amount of energy in the direction equal to the angle of incidence. For contact testing the oscillating crystal is incorporated in a hand held probe, which is applied to the surface of the material to be tested. To facilitate the transfer of energy across the small air gap between the crystal and the test piece, a layer of liquid (referred to as ‘coolant’), usually oil, water or grease, is applied to the surface. As mentioned previously, the crystal does not oscillate continuously but in short pulses, between each of which it is quiescent. Piezo electric materials not only convert electrical pulses to mechanical oscillations, but will also transducer mechanical oscillations into electrical pulses; thus we have not only a generator of sound waves but also a detector of returned pulses. The crystal is in a state to detect returned pulses when it is quiescent. The pulse takes a finite time to travel through the material to the interface and to be reflected back to the probe. The standard method of presenting information in ultrasonic testing is by means of a cathode ray tube, in which horizontal movement of the spot from left to right represents time elapsed. The principle is not greatly different in digitized instruments that have a LCD flat screen. The rate at which the spot moves is such that it gives the appearance of a horizontal line on the screen. The system is synchronized electronically so that at the instant the probe receives its electrical pulse the spot begins to traverse the screen. An upward deflection (peak) of the line on the left hand side of the screen is an indication of this occurrence. This peak is usually termed the initial pulse.
While the base line is perfectly level the crystal is quiescent. Any peaks to the right of the initial pulse indicate that the crystal has received an incoming pulse reflected from one or more interfaces in the material. Since the spot moves at a very even speed across the tube face, and the pulse of ultrasonic waves moves at a very even velocity through the material, it is possible to calibrate the horizontal line on the screen in terms of absolute measurement. The use of a calibration block, which produces a reflection from the back wall a known distance away from the crystal together with variable controls on the flaw detector, allows the screen to be calibrated
in units of distance, and therefore determination of origins of returned pulses obtained from a test piece. It is therefore possible not only to discover a defect between the surface and the back wall, but also to measure its distance below the surface. It is important that the equipment is properly calibrated and, since it is in itself not able to discriminate between intended boundaries of the object under test and unintended discontinuities, the operator must be able to identify the origin of each peak. Further as the pulses form a beam it is also possible to determine the plan position of a flaw. The height of the peak (echo) is roughly proportional to the area of the reflector, though there is on all instruments a control, which can reduce or increase the size of an indication - variable sensitivity in fact. Not only is party of the beam reflected at a material/air interface but also at any junction where there is a velocity change, for example steel/slag interface in a weld. Probing all faces of a test piece not only discovers the three-dimensional defect and measures its depth, but can also determine its size. Two-dimensional (planar) defects can also be found but, unlike radiography, it is best that the incident beam impinges on the defect as near to right angles to the plane as possible.
To achieve this some probes introduce the beam at an angle to the surface. In this manner longitudinal defects in tubes (inner or outer surface) are detected. Interpretation of the indications on the screen requires a certain amount of skill, particularly when testing with hand held probes. The technique is, however, admirably suited to automatic testing of regular shapes by means of a monitor – an electronic device that fits into the main equipment to provide an electrical signal when an echo occurs in a particular position on the trace. The trigger level of this signal is variable and it can be made to operate a variety of mechanical gates and flaw warnings. Furthermore, improvements in computer technology allow test data and results to be displayed and out-putted in a wide variety of formats. Modern ultrasonic flaw detectors are fully solid state and can be battery powered, and are robustly built to withstand site conditions. Since the velocity of sound in any material is characteristic of that material, it follows that some materials can be identified by the determination of the velocity. This can be applied, for example in S.G. cast irons to determine the percentage of graphite Modularity.
Advantages of Ultrasonic Flaw Detection
1. Thickness and lengths up to 30 ft can be tested.
2. Position, size and type of defect can be determined.
3. Instant test results.
4. Portable.
5. Extremely sensitive if required.
6. Capable of being fully automated.
Disadvantages of Ultrasonic Flaw Detection
1. Access to only one side necessary.
2. No consumables.
3. Disadvantages of Ultrasonic Flaw Detection
4. No permanent record available unless one of the more sophisticated test results and data collection systems is used.
5. The operator can decide whether the test piece is defective or not whilst the test is in progress.
6. Indications require interpretation (except for digital wall thickness gauges).
7. Considerable degree of skill necessary to obtain the fullest information from the test.
8. Very thin sections can prove difficult.
Eddy Current and Electro-Magnetic Methods
The main applications of the eddy current technique are for the detection of surface or subsurface flaws, conductivity measurement and coating thickness measurement. The technique is sensitive to the material conductivity, permeability and dimensions of a product. Eddy currents can be produced in any electrically conducting material that is subjected to an alternating magnetic field (typically 10Hz to 10MHz). The alternating magnetic field is normally generated by passing an alternating current through a coil. The coil can have many shapes and can between 10 and 500 turns of wire. The magnitude of the eddy currents generated in the product is dependent on conductivity, permeability and the set up geometry. Any change in the material or geometry can be detected by the excitation coil as a change in the coil impedance The most simple coil comprises a ferrite rod with several turns of wire wound at one end and which is positioned close to the surface of the product to be tested. When a crack, for example, occurs in the product surface the eddy currents must travel farther around the crack and this is detected by the impedance change
Coils can also be used in pairs, generally called a driven pair, and this arrangement can be used with the coils connected differentially. In this way ‘lift off’ (distance of the Probe from the surface) signals can be enhanced.
Coils can also be used in a transformer type configuration where one coil winding is a Primary and one (or two) coil windings are used for the secondary’s.
The detected eddy current signals contain amplitude and phase information and which can be displayed on CRT type displays – non digital displays. Signals can be displayed as the actual, i.e. absolute signal, or with appropriate electronics, only a signal change is displayed. The best results are obtained where only one product parameter is changes, e.g. the presence of a crack.
In practice changes in eddy current signals are caused by differences in composition, hardness, texture, shape, conductivity, permeability and geometry. In some cases the effects of the crack can be hidden by changes in other parameters and unnecessary rejection can occur. However, the coils can be selected for configuration, size and test frequency in order to enhance detection of cracks, conductivity, metal loss etc. as required.
The depth to which the eddy currents penetrate a material can be changed by adjusting the test frequency – the higher the frequency, the lower the penetration; however, the lower the frequency, the lower sensitivity to small defects. Larger coils are less sensitive to surface roughness and vice versa. The latest electronic units are able to operate a wide range of coil configurations in absolute or differential modes and at a wide range of frequencies. For surface testing for cracks in single or complex shaped components, coils with a single ferrite cored winding are normally used. The probe is placed on the component and ‘balanced’ by use of the electronic unit controls. As the probe is scanned across the surface of the component the cracks can be detected. See Fig.1 Where surfaces are to be scanned automatically the single coil windings are suitable only if the lift off distance is accurately maintained. Generally differential coil configurations are used with higher speed scanning systems where lift off effects, vibration effects, etc. can be cancelled out to an acceptable extent. See Fig.2. Tubes, bar and wire can be inspected using an encircling coil and these usually have a coil configuration with one primary and two secondary’s connected differentially. See Fig.3. Most eddy current electronics have a phase display and this gives an operator the ability to identify defect conditions. In many cases signals from cracks, lift off and other parameters can be clearly identified. Units are also available which can inspect a product simultaneously at two or more different test frequencies. These units allow specific unwanted effects to be electronically cancelled in order to give improved defect detection. The eddy current test is purely electrical. The coil units do not need to contact the product surface and thus the technique can be easily automated. Most automated Systems are for components of simple geometry where mechanical handling is simplified.
Advantages of Eddy Current Testing
1. Suitable for the determination of a wide range of conditions of conducting material, such as defect detection, composition, hardness, conductivity, permeability etc. in a wide variety of engineering metals.
2. Information can be provided in simple terms: often go/no go. Phase display electronic units can be used to obtain much greater product information.
3. Extremely compact and portable units are available.
4. No consumables (except probes – which can sometimes be repaired).
5. Flexibility in selection of probes and test frequencies to suit different applications.
6. Suitable for total automation.
Disadvantages of Eddy Current Testing
1. The wide range of parameters which affect the eddy current responses means that the signal from a desired material characteristic, e.g. a crack, can be masked by an unwanted parameter, e.g. hardness change. Careful selection of probe and electronics will be needed in some applications.
2. Generally tests restricted to surface breaking conditions and slightly subsurface flaws.
Visual Inspection
1. Thermo graphic surveys
2. Bore scope and fibre optic inspections
3. Miniature fiberscope inspections form HGP components with complex geometries.
Non-Destructive Testing Methods & Applications
Current NDT methods applied to these ceramic components are expensive, cannot find all the defect of interest, rely on operator interpretations of signals, and frequently use chemicals with deleterious effects on the environment To increase the Probability of Detection (POD) the conventational NDT are advanced by some method are listed as given below:-
1. TOFD Technique (Time of Flight Diffraction).
2. Ultrasonic Phased Array.
3. Process Compensated Resonance Testing (PCRT).
4. Optical NDT Technologies.
TOFD Technique (Time of Flight Diffraction)
The TOFD technique is a fully computerized system able to scan, store, and evaluate indications in terms of height, length, and position with a grade of coverage, accuracy and speed not achieved by other ultrasonic techniques. The TOFD technique is based on diffraction of ultrasonic energy from tips of discontinuities, instead of geometrical reflection on the interface of the discontinuities.
Principle of TOFD
TOFD inspection employs two longitudinal wave (L-wave) angle beam transducers arranged symmetrically opposite facing each other, straddling the weld or base material under test. One probe acts like a transmitter of ultrasonic energy while the other probe receives the ultrasound energy. The transducer, pulser, and amplifier characteristics are selected to generate as broad distribution of energy as possible over the material under test providing full weld coverage. A single-axis scan (that is, along the weld), with a position encoder records the position of the weld and enables the display of digital images in real time.
Capabilities
1. TOFD defect detection does not depend on the defect orientation, in contrast to the pulse echo technique.
2. In contrast to the radiography method, planar defects and cracks, which are not perpendicular to the measured surface, can be detected.
3. Defect height can be exactly determined.
4. Higher POD improves risk reduction and calculation.
Applications
1. New and existing welds
2. Defect monitoring
3. Stress Corrosion Cracking
4. Weld root erosion surveys
Ultrasonic Phased Array
Conventional ultrasonic transducers for NDT commonly consist of either a single active element that both generates and receives high frequency sound waves, or two paired elements, one for transmitting and one for receiving. Phased array probes, on the other hand, typically consist of a transducer assembly with from 16 to as many as 256 small individual elements that can each be pulsed separately. These may be arranged in a strip (linear array), a ring (annular array), a circular matrix (circular array), or a more complex shape. As is the case with conventional transducers, phased array probes may be designed for direct contact use, as part of an angle beam assembly with a wedge, or for immersion use with sound coupling through a water path. Transducer frequencies are most commonly in the range from 2 MHz to 10 MHz. A phased array system will also include a sophisticated computer-based instrument that is capable of driving the multi-element probe, receiving and digitizing the returning echoes, and plotting that echo information in various standard formats. Unlike conventional flaw detectors, phased array systems can sweep a sound beam through a range of refracted angles or along a linear path, or dynamically focus at a number of different depths, thus increasing both flexibility and capability in inspection setups
How do they work?
In the most basic sense, a phased array system utilizes the wave physics principle of phasing, varying the time between a series of outgoing ultrasonic pulses in such a way that the individual wave fronts generated by each element in the array combine with each other to add or cancel energy in predictable ways that effectively steer and shape the sound beam.
This is accomplished by pulsing the individual probe elements at slightly different times. Frequently the elements will be pulsed in groups of 4 to 32 in order to improve effective sensitivity by increasing aperture, which reduces unwanted beam spreading and enables sharper focusing. Software known as a focal law calculator establishes specific delay times for firing each group of elements in order to generate the desired beam shape, taking into account probe and wedge characteristics as well as the geometry and acoustical properties of the test material. The programmed pulsing sequence selected by the instrument's operating software then launches a number of individual wave fronts in the test material. These wave fronts in turn combine constructively and destructively into a single primary wave front that travels through the test material and reflects off cracks, discontinuities, back walls, and other material boundaries like any conventional ultrasonic wave. The beam can be dynamically steered through various angles, focal distances, and focal spot sizes in such a way that a single probe assembly is capable of examining the test material across a range of different perspectives. This beam steering happens very quickly, so that a scan from multiple angles or with multiple focal depths can be performed in a small fraction of a second. The returning echoes are received by the various elements or groups of elements and time-shifted as necessary to compensate for varying wedge delays and then summed. Unlike a conventional single element transducer, which will effectively merge the effects of all beam components that strike its area, a phased array transducer can spatially sort the returning wavefront according to the arrival time and amplitude at each element. When processed by instrument software, each returned focal law represents the reflection from a particular angular component of the beam, a particular point along a linear path, and/or a reflection from a particular focal depth. The echo information can then be displayed in any of several formats.
This is accomplished by pulsing the individual probe elements at slightly different times. Frequently the elements will be pulsed in groups of 4 to 32 in order to improve effective sensitivity by increasing aperture, which reduces unwanted beam spreading and enables sharper focusing. Software known as a focal law calculator establishes specific delay times for firing each group of elements in order to generate the desired beam shape, taking into account probe and wedge characteristics as well as the geometry and acoustical properties of the test material. The programmed pulsing sequence selected by the instrument's operating software then launches a number of individual wave fronts in the test material. These wave fronts in turn combine constructively and destructively into a single primary wave front that travels through the test material and reflects off cracks, discontinuities, back walls, and other material boundaries like any conventional ultrasonic wave. The beam can be dynamically steered through various angles, focal distances, and focal spot sizes in such a way that a single probe assembly is capable of examining the test material across a range of different perspectives. This beam steering happens very quickly, so that a scan from multiple angles or with multiple focal depths can be performed in a small fraction of a second. The returning echoes are received by the various elements or groups of elements and time-shifted as necessary to compensate for varying wedge delays and then summed. Unlike a conventional single element transducer, which will effectively merge the effects of all beam components that strike its area, a phased array transducer can spatially sort the returning wavefront according to the arrival time and amplitude at each element. When processed by instrument software, each returned focal law represents the reflection from a particular angular component of the beam, a particular point along a linear path, and/or a reflection from a particular focal depth. The echo information can then be displayed in any of several formats.
What do the images look like?
In most typical flaw detection and thickness gauging applications, the ultrasonic test data will be based on time and amplitude information derived from processed RF waveforms. These waveforms and the information extracted from them will commonly be presented in one or more of four formats: A-scans, B-scans, C-scans, or S-scans. This section shows some examples of image presentations from both conventional flaw detectors and phased array systems.
A Scan Display
An A-Scan is a simple RF waveform presentation showing the time and amplitude of an ultrasonic signal, as commonly provided by conventional ultrasonic flaw detectors and waveform display thickness gages. An A-scan waveform represents the reflections from one sound beam position in the test piece. The flaw detector A-scan below shows echoes from two side-drilled holes in a steel reference block. The columnar sound beam from a common single-element contact transducer intercepts two out of the three of the holes and generates two distinct reflections at different times that are proportional to the depth of the holes.
A single-element angle beam transducer used with a conventional flaw detector will generate a beam along one angular path. While beam spreading effects will cause the beam diameter to increase with distance, the area of coverage or field of vision of a conventional angle beam will still basically be limited to one angular path. In the example below, a 45 degree wedge at one fixed position is able to detect two of the side-drilled holes in the test block because they fall within its beam, but it is not possible to detect the third without moving the transducer forward. A phased array system will display similar A-scan waveforms for reference, however in most cases they will be supplemented by B-scans, C-scans, or S-scans as seen below. These standard imaging formats aid the operator in visualizing the type and position of flaws in a test piece
B Scan display
A B-scan is an image showing a cross-sectional profile through one vertical slice of the test piece, showing the depth of reflectors with respect to their linear position. B-scan imaging requires that the sound beam be scanned along the selected axis of the test piece, either mechanically or electronically, while storing relevant data. In the case below, the B-scan shows two deep reflectors and one shallower reflector, corresponding to the positions of the side drilled holes in the test block. With a conventional flaw detector, the transducer must be moved laterally across the test piece.
C-Scan displays
A C-scan is a two dimensional presentation of data displayed as a top or planar view of a test piece, similar in its graphic perspective to an x-ray image, where color represents the gated signal amplitude at each point in the test piece mapped to its x-y position. With conventional instruments, the single-element transducer must be moved in an x-y raster scan pattern over the test piece. With phased array systems, the probe is typically moved physically along one axis while the beam electronically scans along the other. Encoders will normally be used whenever precise geometrical correspondence of the scan image to the part must be maintained, although unencoded manual scans can also provide useful information in many cases.
The images that follow show C-scans of a reference block made with a conventional immersion scanning system with a focused immersion transducer, and with a portable phased array system using an encoded hand scanner and a linear array. While the graphic resolution is not fully equivalent, there are other considerations. The phased array system is field portable, which the conventional system is not, and costs about one-third the price. Additionally, the phased array image was made in a few seconds, while the conventional immersion scan took several minutes.
The images that follow show C-scans of a reference block made with a conventional immersion scanning system with a focused immersion transducer, and with a portable phased array system using an encoded hand scanner and a linear array. While the graphic resolution is not fully equivalent, there are other considerations. The phased array system is field portable, which the conventional system is not, and costs about one-third the price. Additionally, the phased array image was made in a few seconds, while the conventional immersion scan took several minutes.
S-Scan displays
An S-scan or sectorial scan image represents a two-dimensional cross-sectional view derived from a series of A-scans that have been plotted with respect to time delay and refracted angle. The horizontal axis corresponds to test piece width, and the vertical axis to depth. This is the most common format for medical sonograms as well as for industrial phased array images. The sound beam sweeps through a series of angles to generate an approximately cone-shaped cross-sectional image. It should be noted that in this example, by sweeping the beam the phased array probe is able to map all three holes from a single transducer position.
Where is phased array systems used?
Ultrasonic phased array systems can potentially be employed in almost any test where conventional ultrasonic flaw detectors have traditionally been used. Weld inspection and crack detection are the most important applications, and these tests are done across a wide range of industries including aerospace, power generation, petrochemical, metal billet and tubular goods suppliers, pipeline construction and maintenance, structural metals, and general manufacturing. Phased arrays can also be effectively used to profile remaining wall thickness in corrosion survey applications.
The benefits of phased array technology over conventional UT come from its ability to use multiple elements to steer, focus and scan beams with a single transducer assembly. Beam steering, commonly referred to sectorial scanning, can be used for mapping components at appropriate angles. This can greatly simplify the inspection of components with complex geometries. The small footprint of the transducer and the ability to sweep the beam without moving the probe also aids inspection of such components in situations where there is limited access for mechanical scanning. Sectorial scanning is also typically used for weld inspection. The ability to test welds with multiple angles from a single probe greatly increases the probability of detection of anomalies. Electronic focusing permits optimizing the beam shape and size at the expected defect location, thus further optimizing probability of detection. The ability to focus at multiple depths also improves the ability for sizing critical defects for volumetric inspections. Focusing can significantly improve signal-to-noise ratio in challenging applications, and electronic scanning across many groups of elements allows for C-Scan images to be produced very rapidly.
The benefits of phased array technology over conventional UT come from its ability to use multiple elements to steer, focus and scan beams with a single transducer assembly. Beam steering, commonly referred to sectorial scanning, can be used for mapping components at appropriate angles. This can greatly simplify the inspection of components with complex geometries. The small footprint of the transducer and the ability to sweep the beam without moving the probe also aids inspection of such components in situations where there is limited access for mechanical scanning. Sectorial scanning is also typically used for weld inspection. The ability to test welds with multiple angles from a single probe greatly increases the probability of detection of anomalies. Electronic focusing permits optimizing the beam shape and size at the expected defect location, thus further optimizing probability of detection. The ability to focus at multiple depths also improves the ability for sizing critical defects for volumetric inspections. Focusing can significantly improve signal-to-noise ratio in challenging applications, and electronic scanning across many groups of elements allows for C-Scan images to be produced very rapidly.
Process Compensated Resonance Testing (PCRT)
PCRT is an evolution of Resonant Ultrasound Spectroscopy (RUS), the analysis of the resonant frequencies of a part to detect flaws. Developed by Los Alamos Nationals Lab and covered by ASTM Stand Guide E2001-08, RUS is based on the physics fundamental that rigid parts resonate at specific frequencies that are a function of mass, geometry, and material properties. PCRT combines RUS with proprietary pattern recognition algorithms, based on Mahalanobis- Taguchi System (MTS) statistical analysis, to identify the resonance spectra characteristics that represent acceptable parts and those caused by unacceptable defects. The operator is presented with a clear Pass/Fail result that requires no interpretation. This eliminates operator subjectivity and the need for expertise in analyzing the test results, enabling full automation. PCRT is the only NDT technology that can effectively compare the results of successive inspections to detect the accumulation of fatigue and damage.
Figure 1 shows examples of selected resonances for acceptable and unacceptable parts. The upper spectrum is a ceramic ball with no defects. The lower spectrum is for a ball with a C-spall crack as indicated by the resonant peak split. The structural defect in the part is directly reflected in the resonant spectra.
The PCRT System consists of a control computer, a transceiver, and a component nest. The computer, loaded with the PCRT software, provides the user interface and communicates with the transceiver. The transceiver is a precision signal generator and spectrum analyzer. The nest holds three lead-zirconate-titantate (PZT) contact transducers that interface with the part. One transducer excites the part while the other two measure the part’s resonant response. A PCRT system diagram is shown in Figure 3, and sample hardware is shown in Figure 4. The nest shown is for a ceramic ball. Testing a part is simple. The part is placed on the nest, the part temperature is recorded (so the software can compensate for frequency variation vs. temperature), and the testing software is run. Tests require no part preparation and are typically completed in seconds, yielding superior throughput and cost-effectiveness.
Optical NDT Technologies
Optical fibre NDT features the use of optical fibres to collect and sense the signal light from the
object under test (OUT). The optical fibre is resistant to electromagnetic interference and corrosion, which means that in can be used in extreme environments. The optical fibre is small and lightweight and could be either attached to the surface of the tested structure or be embedded inside, having little impact on the structure itself. Fibre optic sensors can be designed to measure or sense just about anything. A few examples include liquid level, chemical, pressure, electric field, vibration strain and temperature. There are many types of sensor designs, including Bragg, IFPI (Intrinsic Fabry Perot Interferometer) and EFPI (Extrinsic Fabry Perot Interferometer) [4]. While there are many types of optical sensors, the focus of this section is on the fibre Bragg grating sensor. When the physical quantities to be measured are strain, temperature, pressure, etc., the optical fibre itself can be the sensor. In these cases, the physical quantities to be measured can cause a change of the intensity, phase, polarization, wavelength, or transit time of the light in the fibre system, and NDT is implemented through monotoring these optical parameters.Light intensity monitoring is the simplest and most frequently used technology in practice. The most successful application of optical fibre NDT is the field of civil engineering.
Working
The optical setup for the polarization-based fibre optic sensor. It is formed by polarizing the light from a light source (polarized He-Ne laser) via a half-wave plate. The half wave plate here is used to rotate the polarization state of the plane polarized He-Ne light. That could be a length of polarization-preserving fibre. The fibre here serves as the sensing medium. Under external perturbation such as stress or strain, the phase difference between two polarization states is changed, and so the output polarization state is changed accordingly. Hence, by using PC analyzing the output polarization state at the exit end of the fibre, the external perturbation can be detected.
Fig(18)A structure of optical fiber polarization sensor detecting system
Optical fibre sensing is usually used as a non-destructive testing method at multiple locations for
long time monitoring, for single or multiple parameters like temperature and strain. To monitor the status of a large object or system, like a natural gas pipeline, multiple discrete optical fiber sensors are placed at different locations of the object or system, forming a distributed sensor network. Real-time parameters like temperature and strain are collected by the sensors and transmitted to a data processing center through an optical transmission network. This method uses the optical fibre as the sensing medium to monitor more than one physical parameters, as well as the transmission medium to combine the distributed sensor network. It has advantages of real-time monitoring, large data processing capability, high-integration, and excellent environment tolerance. Therefore, distributed fibre sensing technology is a hot research area today, and the multi-sensor network addressing (or multiplexing) and location (or demodulation) methods are important research fields in NDT.
No comments:
Post a Comment
leave your opinion