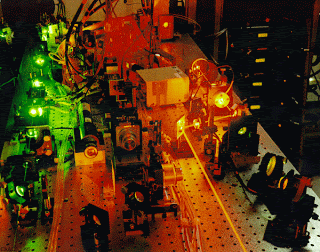
ABSTRACT:
This paper introduces photonic crystal fiber uses devices. The modern instruments and techniques are costly as well as out of reach from rural areas. Photonic crystal fiber is now finding applications in fiber optic communications, fiber lasers, nonlinear devices, high power transmission ,highly sensitive gas sensors.
INTRODUCTION:
Photonic crystal:- Photonic crystals are periodic optical nanostructures that are designed to affect the motion of photons in a similar way that periodicity of a semiconductor crystal affects the motion of electrons. Photonic crystals occur in nature and in various forms have been studied scientifically for the last 100 years. Photonic crystals are composed of periodic dielectric or mettalo-dielectric nanostructures that affect the propagation of electromagnetic waves (EM) in the same way as the periodic potential in a semiconductor crystal affects the electron motion by defining allowed and forbidden electronic energy bands. Essentially, photonic crystals contain regularly repeating internal regions of high and low dielectric constant. Photons (behaving as waves) propagate through this structure - or not - depending on their wavelength. Wavelengths of light that are allowed to travel are known as modes, and groups of allowed modes form bands. Disallowed bands of wavelengths are called photonic band gaps. This gives rise to distinct optical phenomena such as inhibition of spontaneous emission, high-reflecting omni-directional mirrors and low-loss-waveguide, amongst others.
Since the basic physical phenomenon is based on diffraction, the periodicity of the photonic crystal structure has to be of the same length-scale as half the wavelength of the EM waves i.e. ~350 nm (blue) to 700 nm (red) for photonic crystals operating in the visible part of the spectrum - the repeating regions of high and low dielectric constants have to be of this dimension. This makes the fabrication of optical photonic crystals cumbersome and complex.
Applications:
Photonic crystals are attractive optical materials for controlling and manipulating the flow of light. One dimensional photonic crystal are already in widespread use in the form of thin-film optics with applications ranging from low and high reflection coatings on lenses and mirrors to colour changing paints and inks. Higher dimensional photonic crystals are of great interest for both fundamental and applied research, and the two dimensional ones are beginning to find commercial applications. The first commercial products involving two-dimensionally periodic photonic crystals are already available in the form of photonic-crystal fibers, which use a micro scale structure to confine light with radically different characteristics compared to conventional optical fiber for applications in nonlinear devices and guiding exotic wavelengths. The three-dimensional counterparts are still far from commercialization but offer additional features possibly leading to new device concepts (e.g. optical computers), when some technological aspects such as manufacturability and principal difficulties such as disorder are under control.
Photonic crystal fiber:
Photonic-crystal fiber (PCF) is a new class of optical fiber based on the properties of photonic crystals. Because of its ability to confine light in hollow cores or with confinement characteristics not possible in conventional optical fiber, PCF is now finding applications in fiber-optic communications, fiber lasers, nonlinear devices, high-power transmission, highly sensitive gas sensors, and other areas.
Construction:
Generally, such fibers are constructed by the same methods as other optical fibers: first, one constructs a "preform" on the scale of centimeters in size, and then heats the preform and draws it down to a much smaller diameter (often nearly as small as a human hair), shrinking the preform cross section but (usually) maintaining the same features. In this way, kilometers of fiber can be produced from a single preform. The most common method involves stacking although drilling/milling was used to produce the first aperiodic designs. This formed the subsequent basis for producing the first soft glass and polymer structured fibers.
Most photonic crystal fibers have been fabricated in silica glass, but other glasses have also been used to obtain particular optical properties (such has high optical non-linearity). There is also a growing interest in making them from polymer, where a wide variety of structures have been explored, including graded index structures, ring structured fibers and hollow core fibers. These polymer fibers have been termed "MPOF", short for microstructured polymer optical fibers(van Eijkelenborg, 2001). A combination of a polymer and a chalcogenide glass was used by Temelkuran et al. (2002) for 10.6 µm wavelengths (where silica is not transparent).
Modes of operation:
Photonic crystal fibers can be divided into two modes of operation, according to their mechanism for confinement. Those with a solid core, or a core with a higher average index than the microstructured cladding, can operate on the same index-guiding principle as conventional optical fiber — however, they can have a much higher effective- refractive index contrast between core and cladding, and therefore can have much stronger confinement for applications in nonlinear optical devices, polarization-maintaining fibers, (or they can also be made with much lower effective index contrast). Alternatively, one can create a "photonic band-gap" fiber, in which the light is confined by a photonic band-gap created by the micro-structured cladding – such a band-gap, properly designed, can confine light in a lower-index core and even a hollow (air) core. Band-gap fibers with hollow cores can potentially circumvent limits imposed by available materials, for example to create fibers that guide light in wavelengths for which transparent materials are not available (because the light is primarily in the air, not in the solid materials). Another potential advantage of a hollow core is that one can dynamically introduce materials into the core, such as a gas that is to be analyzed for the presence of some substance. PCF can also be modified by coating the holes with sol-gels of similar or different index material to enhance its transmittance of light.
Photonic Crystal Waveguides Based on
An Anti-resonant Reflecting Platform:
The trend of miniaturising optical integrated contours has been boosted by the concept of photonic band gap (PBG) materials, in which cavities and waveguides with novel properties can be created by introducing point like or line defect states in the photonic crystal lattice. However, there are certain obstacles on the way towards the integrated circuit based on PBG structures. Specifically, propagation losses in photonic crystal waveguides (PCWs) are too high in comparison to rib or ridge waveguides. Thus, the reduction of losses in straight PCWs is a key topic on the way of designing integrated photonic circuits based thoroughly on PBG elements. Several designs addressing the high propagation losses of PCWs have been suggested. Here we propose an alternative, namely anti-resonant reflecting optical waveguides (ARROW) concept to SOI-based PCWs to reduce the out-of-plane scattering losses.
APPLICATION OF THE ARROW CONCEPT IN PHOTONIC CRYSTAL WAVEGUIDES:
The thickness of the first silicon cladding beneath the silica core is deliberately chosen to provide high reflectance by the interference principle, like a Fabry-Perot resonator, which have broad reflecting windows on antiresonant wavelengths and sharp transmission peaks on resonant wavelengths. In order to enhance the resonance effect the silicon cladding is underpinned by a second cladding layer made from silica. The condition for resonant reflection of the evanescent fields penetrating through the first cladding is fulfilled by making the thickness of the silicon cladding approximately half that of the silica core. Thus, in total, a reflection around 99.96% at the bottom interface of the core arises. Under the zero-order approximation, the claddings are nothing else but λ/4 plates with respect to the transverse component of the wave-vector. An attempt to apply the ARROW concept with PCWs has recently been made, but the authors chose the core thickness to be 7 microns to improve the Coupling between PCWs and fibers. However, etching 7 microns deep holes with smooth sidewalls is a very Challenging problem.
The original ARROW idea was further developed. where new generations of ARROW structures with a high index core and a low index first cladding were proposed and characterized. These are the so-called ARROW-B (Fig.1, b) and ARROW-C configurations, which are applicable for SOI-based PCWs.
One significant issue has to be stressed concerning ARROW-B based PCWs. A standard core thickness of Classical ARROW structures is about 4-5 microns or more, and losses rapidly decrease with increasing Core thickness. On the contrary, the thickness of the silicon core in an SOI-based PCW is severely limited by the Requirement of a reasonable size of the photonic band gap. The gap-midgap frequency ratio was analyzed in Paper and it was found that the optimal slab thickness for photonic crystal structures defined by a triangular Lattice of holes is 0.7a, where a is the lattice constant. If the slab thickness is increased the gap-mid-gap Frequency ratio is decreased. Keeping in mind that promising applications of PCWs such as submicron-radii Bends, splitters, and couplers are totally dependent on the PBG effect; we have to restrict ourselves to the case of The ~ 0.7a thick silicon core, which inevitably triggers the propagation loss to increase. The main purpose of our Investigation is to verify the usefulness of the ARROW concept in order to decrease the propagation losses in SOI-based PCWs.
Characterisation of Arrow- B photonic crystal waveguides:-
There is a standard procedure for direct calculation of propagation losses using numerical techniques .
High-Power Photonic Crystal Fiber Lasers:
One of the fastest growing and most promising applications of optical fiber is in high-power fiber lasers. In the past few years, fiber lasers have evolved from low-power systems for niche applications into challengers to the traditional high-power industrial lasers for processing. Fiber lasers are gaining market share at a strong pace and are projected to be the industry standard within a few years for printing.cutting,welding and marking.
Their advantages are low operating costs, high beam quality and high efficiency in a maintenance-free format with a small footprint and low weight. In particular, they offer a superior beam quality compared with other laser systems for power levels in the kilowatt range. This improves precision and processing speeds for industrial materials processing systems and introduces the possibility for achieving extreme power levels by combining multiplelasers.
The highest reported power level for a single-mode fiber laser is approximately 3 kW, demonstrated by IPG Photonics Corp. of Oxford, Mass. It is worth noting that the record power level has increased dramatically over a very short time and further increases no doubt lie ahead.
Fiber laser concepts:
Photonic crystal fiber is perhaps the most promising technology to significantly lift the current power levels. This is because of its increased flexibility in single-mode core sizes, the increased numerical aperture of pump cores in double-clad fiber configurations and the high thermal stability of low-loss all-glass structures.
A double-clad fiber laser converts low-beam-quality pump light into laser light with high beam quality.
A fiber laser is based on a rare-earth-doped fiber that serves as a gain medium. An optical pump source typically, for high-power applications, in the form of multimode laser diodes provides energy for the gain medium. The active fiber converts the low-beam-quality pump light into signal light with high beam quality.
Typically, double-clad fiber designs are employed, where light from the laser diodes is coupled into a multimode cladding (also referred to as the pump core) surrounding the active single-mode signal core. Traditional active fiber, such as that used for amplifiers in telecommunications systems, basically is a standard transmission fiber in which the core region has been doped with rare-earth ions. Because the pump light must be coupled into the small signal core, this type of fiber preferably is pumped with single-mode pump lasers. The power limitations of single-mode pump sources make the fiber unsuitable for high-power applications. The primary advantage of the double-clad design is the result of the large pump area and high numerical aperture, enabling pumping with relatively low-cost multimode-stacks.
The pump light can be coupled by free-space optics such as lenses and mirrors or can be delivered in one or more multimode fibers that are fused onto the active fiber. The preferred gain medium for high-power lasers is ytterbium, a result of its high efficiency: Approximately 80 percent of the pump light can be converted to the signal light. The laser cavity is formed using free-space mirrors, fiber Bragg gratings, the fiber’s facets or a combination of these.
Fiber lasers can be simple oscillators in which Bragg reflectors (shown here), fiber facets or external mirrors define the resonator (a). Alternatively, they may be configured as master oscillator power amplifiers in which a signal from a seed laser is amplified in a power amplifier (b).
When scaling fiber lasers to higher powers, it is important to optimize the fiber for the efficient coupling of the pump light, reduction of nonlinear effects, high conversion of pump light and good thermal properties. In short, desirable features of the fiber are a high numerical aperture for the pump light, a large core size, a short fiber length, a low thermal load and high temperature resistance, and a low residual pump power.
The high numerical aperture enables a reduction in the size of the pump core and thereby an increase in pump absorption. High pump absorption is important to ensure short fiber lengths, which are desirable to minimize the length-dependent nonlinear effects as well as to reduce residual pumping and to increase the efficiency of the system. Moving to larger signal cores further increases the pump absorption and lowers the intensity in the core, suppressing nonlinear effects.
Standard fibers are limited in both single-mode core size and pump-core numerical aperture, however, which ultimately limits their use in high-power applications. Photonic crystal fiber technology provides a means to bypass these limitations and to realize fibers optimized for high-power operation.
Commercial holey fiber laser:
By combining the active air-clad fiber with specially designed pump combiners, laser and amplifier subassemblies can be created based on photonic crystal fiber that can be readily pumped with standard industry diode lasers. The first such commercial laser, intended for applications such as marking and low-power welding, is the aeroLase-20 from Crystal Fiber A/S of Bkerødir, Denmark.
The aeroLase-20, the first commercial all-fiber laser based on photonic crystal fiber, is a simple fiber oscillator with Bragg gratings.
the 10 × 3 × 22-cm instrument comprises a single-mode ytterbium-doped air-clad fiber with a complete Bragg cavity, a standard 7:1 pump combiner and a step-index delivery fiber. The system is designed to be pumped with seven fiber-coupled single-emitter diodes in 100-μm 0.22-NA fibers.
When pumped with seven 915-nm, 5-W diodes, the output from the aeroLase-20 is more than 22 W, delivered in a conventional single-mode fiber. The slope efficiency is 74 percent, and the total optical-to-optical efficiency is more than 64 percent, resulting in a wall-plug efficiency of more than 30 percent. Testing shows a drop in the output power of less than 10 percent over 1000 hours, including pump diode degradation. The output wavelength is 1075 nm, and the line width at full power is less than 200 pm.
CONCLUSIONS:
I have addressed an anti-resonant reflection wave-guiding platform to be employed within PCW designs. A strong resonance dependence of the transmission spectrum is clearly seen. Structures with a lowered core index have larger losses .we also checked the roll of photonic crystal fiber in high power laser. I also describe the Fiber laser concepts and Commercial holey fiber laser.
No comments:
Post a Comment
leave your opinion